Wildfires are climate risks that threaten the forests’ capacity to store carbon. Recently, California has set forest management goals to ease these risks. However, the current management strategies can incur carbon losses. This article looks at a study in Proceedings of the National Academy of Sciences of the United States of America detailing a systems approach to evaluate climate change mitigation potential and wildfire outcomes throughout forest management scenarios and various wood products.
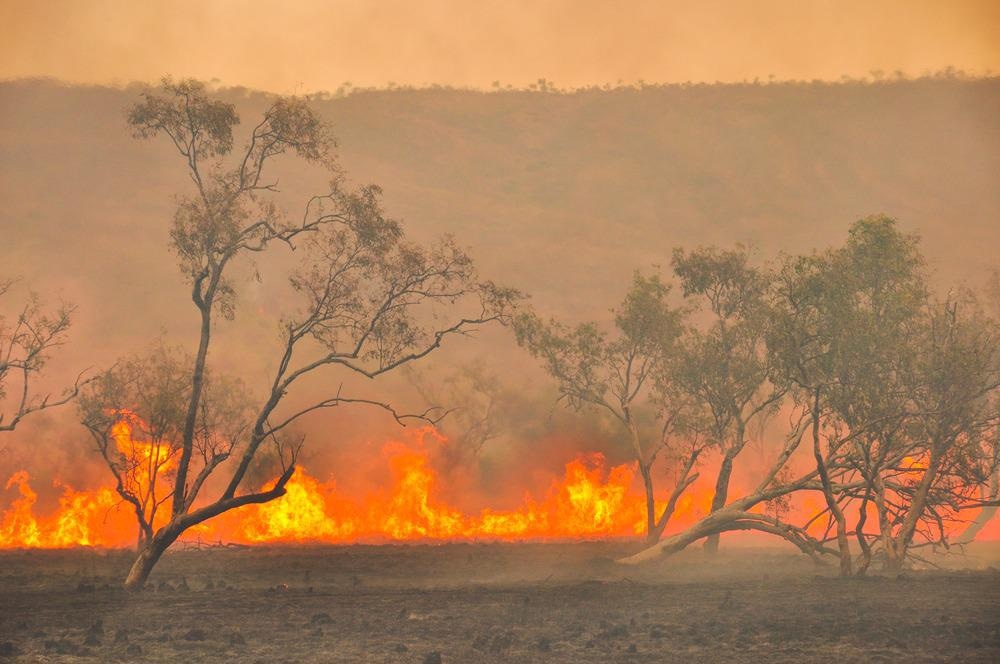
Image Credit: John Crux/Shutterstock.com
Climate change poses a major challenge, particularly in managing temperate forests in California. The California forests are denser, younger and are vulnerable to disturbances such as wildfire, drought, disease, and insects. California forests experience risk from fire severity and frequency, which is a threat to forest ecosystems and their capacity to sequester carbon. These wildfire events are mostly because of climate change.
However, some recent investigations have shed light on a new aspect - the ability of forests to help meet climate goals. Even though uncertainties exist on how forest treatment affects net carbon emissions, it is considered that more effective use of harvested wood can enhance the carbon balance of management. However, diverse wood products vary based on substitution benefits, production emissions, and end-of-life emissions.
The increasing wildfire risk led California’s Forest Climate Action Team as well as the State of California to set a goal to decrease wildfire hazard on 1 million (M) acres (0.4 M ha) per year. The plan led to many different strategies such as fuel reduction treatments, expanded use of harvested wood products, and timber harvest.
But most of these treatments were expensive and most are burned after treatment, leading to carbon release. This article proposes another alternative to managing multiple goals. The current study analyzes how a powerful market for forest residues affects forest treatment in California.
The authors initially modeled forest health-oriented thinning treatments and the wildfire outcomes on California’s private and public timberland with Forest Inventory and Analysis (FIA) data (see Figure 1).
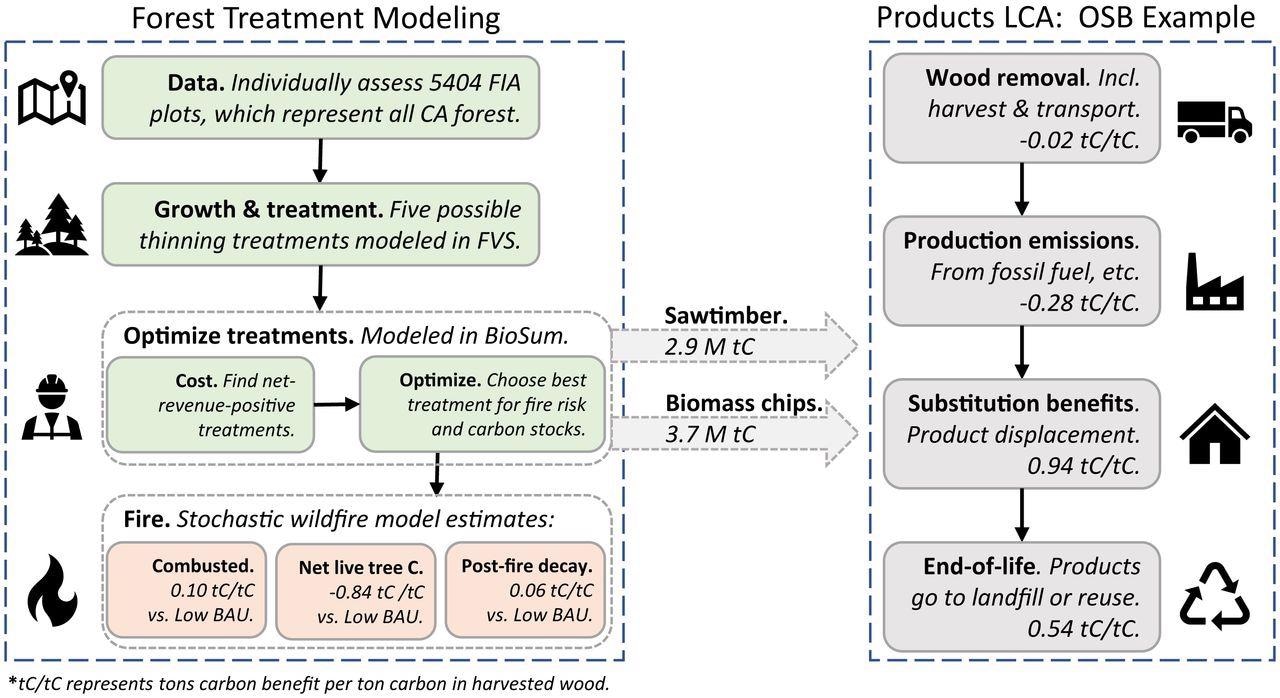
Figure 1. Modeling framework, system boundaries, and example results for one product, oriented strand board (OSB). Product carbon benefits (Right) are specific to OSB, while in-forest carbon fluxes (Left) are common to all products in the IWP scenario. Carbon benefit values presented are cumulative over 40 years. Image Credit: Cabiyo, et al., 2021
Three management scenarios were considered and later the carbon benefits of various pathways for harvested wood were examined. Figure 2 represents the net carbon balance from forest management and wood product markets in California.
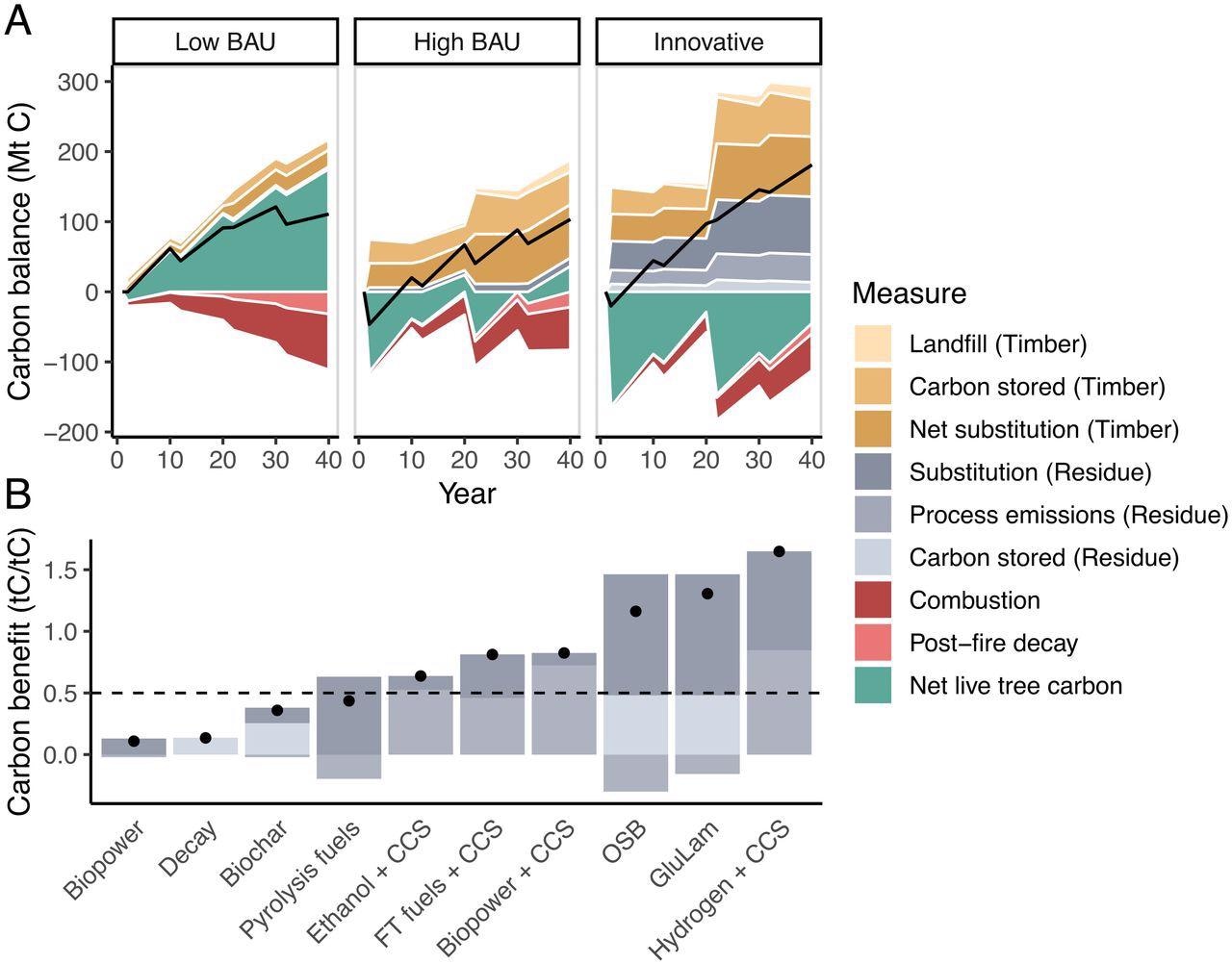
Figure 2. Life cycle, forest carbon balance across (A) three scenarios and (B) several technology pathways. Net carbon values are represented by dots in B and black lines in A. In B, the dotted line represents the threshold used to select the suite of technologies in IWP. Net live-tree carbon values are relative to carbon stocks in year zero, and large decreases are associated with harvest events. In Low BAU, we model management only on corporate land that was potentially profitable (net revenue > $2,500/ha). In High BAU, we model management wherever it is net revenue positive with a delivered residue price of $0. In Innovative (IWP), we model management wherever it is net revenue positive with a delivered residue price of up to $100/ODT. The treatment area under IWP defines the study area for High and Low BAU, which includes untreated forest. Image Credit: Cabiyo, et al., 2021
Results
The Business as Usual with Limited Management (Low BAU) scenario portrays a low-management future. Both high rates of carbon storage and high fire hazard characterized this scenario in the untreated forest.
Business as Usual with Expanded Management (High BAU) scenario, considers the impact of increasing the scale of management without subsidy and without the revenue from forest residues. The harvested saw timber is almost three times bigger than in Low BAU and most of the woods are from smaller trees (see Figure 3).
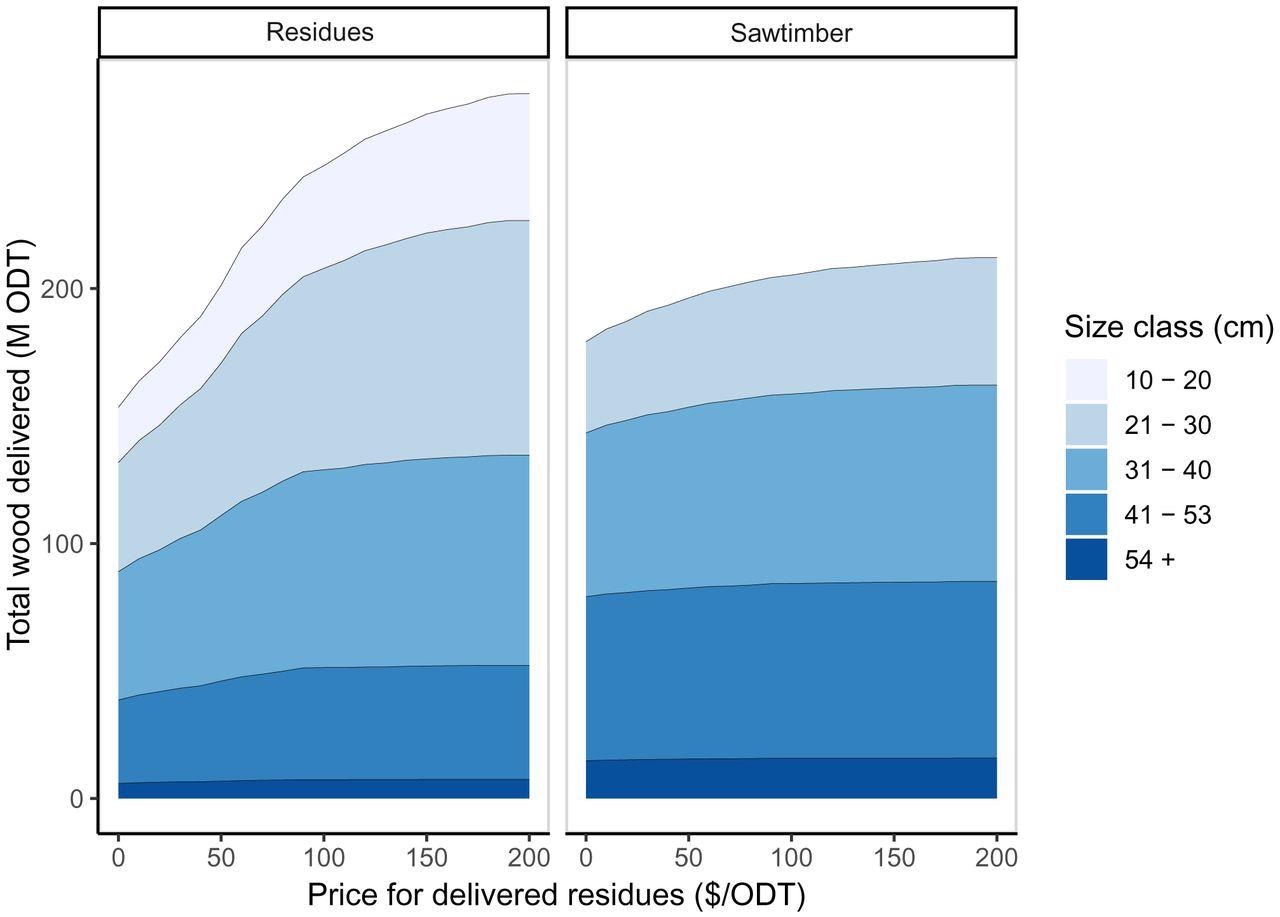
Figure 3. Wood availability at increasing delivered forest residue prices by DBH class. Residues include small trees, tops, and branches from larger trees harvested for sawtimber, and entire trees of noncommercial species. Image Credit: Cabiyo, et al., 2021
The High BAU scenario decreases wildfire hazard on more hectares compared to Low BAU. The Innovative Wood Products (IWP) scenario investigates how the inventive uses of forest residues facilitate better carbon and economic outcomes from management. In IWP, 1.3 M ha (3.1 M ac) could be treated in all the areas treated. Figure 4 shows the fire hazard reduction in an IWP scenario.
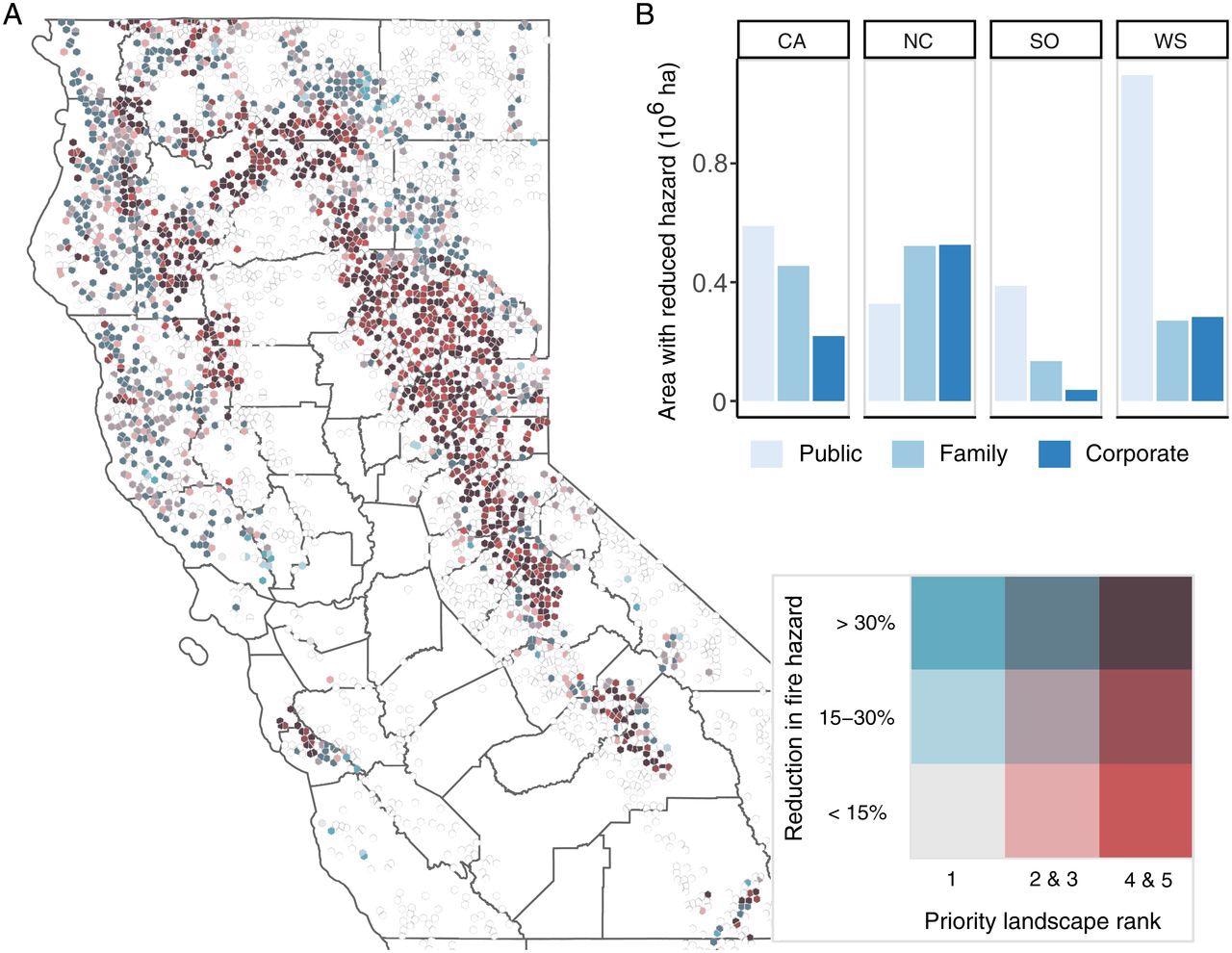
Figure 4. Fire hazard reduction in the IWP scenario in (A) CalFire Fire Priority Zones and (B) summed across the study area. Reduction in fire hazard is defined as the difference in basal area mortality fraction with and without treatment in the event of a wildfire with severe fire weather. In B, each hexagon represents a single FIA plot, which is statistically representative of a larger area of forest (usually ∼2,000 to 2,500 ha). Empty hexagons represent untreated plots, and county boundaries are shown in the background. In B, values are grouped by FVS Variants, where CA is Central California, NC is North Coast, SO is Northeast California, and WS is Western Sierra. Colors represent ownership groups, in which “Family” is noncorporate private land. Image Credit: Cabiyo, et al., 2021
The carbon benefits differ substantially (see Figure 2B) for forest residue products, with biopower being the most widely used one. Technologies harboring a large fraction of carbon storage have the greatest benefits.
The forest sector is a net carbon sink in all three scenarios. The Low BAU and High BAU had similar net carbon benefits, while the IWP scenario has a larger carbon benefit. Conventional saw timber products play a vital role in supporting a positive net carbon balance of management.
However, the IWP scenario shows obvious benefits from the innovative use of forest residues and saw timber. The innovative wood use might be crucial in achieving California’s dual goals of decreasing both wildfire hazards and CO2 emissions.
Discussion
The treatments employed enhance multiple ecosystem benefits. This article concludes that carbon-beneficial treatment is not possible without including wood products. Innovative wood use ensures that wildfire-motivated treatments provide climate benefits.
In California, innovative wood use has two major value propositions—revenue increase from harvested wood and enhancing carbon balance of forest management. Climate policies also play a significant role in such cases.
Limitations
The current study presented Low BAU and High BAU as baseline scenarios, however, both these scenarios are not a perfect representation of reality. These scenarios were used as they closely approximate the present state of forest management in California.
The research used an attributional life cycle analysis (LCA) approach. Moreover, the model forest growth in the Forest Vegetation Simulator (FVS), overestimates growth and underrates mortality. This article did not model the effect of non-fire climate effects and the persistent shifts in vegetation.
Methodology
The FIA BioSum modeling framework was employed to comprehend the management outcomes on California timberland. Data were gathered from 5,404 field-sampled FIA plots and the forests were classified. Five forest treatments were stimulated for each FIA plot. A “Grow Only” alternative was also stimulated to represent the untreated forest. The costs and values were evaluated using BioSum.
Potential fire outcomes were modeled for every year and a stochastic model was developed. The study also simulated both 90th and 97.5th percentile fire weather conditions linked with wildfire occurrence in California forests.
The study depended on data from research works using the Regulated Emissions, Greenhouse Gases, and Energy Use in Transportation (GREET) model. For saw timber, a methodology from prior research work was adapted.
Conclusion
The results indicate that effective wood use plays a vital role. IWP can increase the scale of management and carbon benefits from forest residues, which would advance current forest management and climate goals in California. Even though the article integrates various crucial elements of a complex system, there were significant limitations.
Journal Reference:
Cabiyo, B., Fried, J. S., Collins, B. M., Stewart, W., Wong, J., Sanchez, D. L. (2021) Innovative wood use can enable carbon-beneficial forest management in California. Proceedings of the National Academy of Sciences of the United States of America, 118(49), p. e2019073118. Available online: https://www.pnas.org/content/118/49/e2019073118#F4.
References and Further Reading
- McDowell, N. G., et al. (2020) Pervasive shifts in forest dynamics in a changing world. Science, 368, p. eaaz9463. doi.org/10.1126/science.aaz9463.
- Anderegg, W. R. L., et al. (2020) Climate-driven risks to the climate mitigation potential of forests. Science, 368, eaaz7005. doi.org/10.1126/science.aaz7005.
- Lydersen, J M & Collins, B M (2018) Change in vegetation patterns over a large forested landscape based on historical and contemporary aerial photography. Ecosystems (N. Y.), 21, pp. 1348–1363. doi.org/10.1007/s10021-018-0225-5.
- McIntyre, P. J., et al. (2015) Twentieth-century shifts in forest structure in California: Denser forests, smaller trees, and increased dominance of oaks. Proceedings of the National Academy of Sciences of the United States of America, 112, pp. 1458–1463. doi.org/10.1073/pnas.1410186112.
- Westerling, A. L., et al. (2007) Climate change and wildfire in California. Climate Change, 87, pp. 231–249. doi.org/10.1007/s10584-007-9363-z.
- Abatzoglou, J. T., et al. (2016) Impact of anthropogenic climate change on wildfire across western US forests. Proceedings of the National Academy of Sciences of the United States of America, 113, pp. 11770–11775. doi.org/10.1073/pnas.1607171113.
- Mann, M. L., et al. (2016) Incorporating anthropogenic influences into fire probability models: Effects of human activity and climate change on fire activity in California. PLoS ONE, 11, p. e0153589. doi.org/10.1371/journal.pone.0153589.
- Stevens-Rumann, C. S., et al. (2018) Evidence for declining forest resilience to wildfires under climate change. Ecology Letters, 21, pp. 243–252. doi.org/10.1371/journal.pone.0153589.
- Stevens, J. T., et al. (2017) Changing spatial patterns of stand-replacing fire in California conifer forests. Forest Ecology and Management, 406, pp. 28–36. doi.org/10.1016/j.foreco.2017.08.051.
- Liang, S., et al. (2018) Large-scale restoration increases carbon stability under projected climate and wildfire regimes. Frontiers in Ecology and the Environment, 16, pp. 207–212. doi.org/10.1016/j.foreco.2017.08.051.
- Davis, K. T., et al. (2019) Wildfires and climate change push low-elevation forests across a critical climate threshold for tree regeneration. Proceedings of the National Academy of Sciences of the United States of America, 116, pp. 6193–6198. doi.org/10.1073/pnas.1815107116.
- Starrs, C. F., et al. (2018) The impact of land ownership, firefighting, and reserve status on fire probability in California. Environmental Research Letters, 13, p. 034025. doi.org/10.1088/1748-9326/aaaad1.
- Christensen, G. A., et al. (2019) AB 1504 California forest ecosystem and harvested wood product carbon inventory: 2017 reporting period: Final report (California Department of Forestry; Fire Protection.
- Bastin, J. F., et al. (2019) The global tree restoration potential. Science, 365, pp. 76–79. doi.org/10.1126/science.aax0848.
- Griscom, B W., et al. (2017) Natural climate solutions. Proceedings of the National Academy of Sciences of the United States of America, 114, pp. 11645–11650. doi.org/10.1073/pnas.1710465114.
- Fargione, J. E., et al. (2018) Natural climate solutions for the United States. Science Advances, 4, p. eaat1869. https://doi.org/10.1126/sciadv.aat1869.
- Cameron, D. R., et al. (2017) Ecosystem management and land conservation can substantially contribute to California’s climate mitigation goals. Proceedings of the National Academy of Sciences of the United States of America, 114, pp. 12833–12838. doi.org/10.1073/pnas.1707811114.
- Law, B. E., et al. (2018) Land use strategies to mitigate climate change in carbon dense temperate forests. Proceedings of the National Academy of Sciences of the United States of America, 115, pp. 3663–3668. doi.org/10.1073/pnas.1720064115.
- Sleeter, B. M., et al. (2019) Effects of 21st-century climate, land use, and disturbances on ecosystem carbon balance in California. Global Change Biology, 25, pp. 3334–3353. doi.org/10.1111/gcb.14677.
- Hudiburg, T. W., et al. (2019) Meeting GHG reduction targets requires accounting for all forest sector emissions. Environmental Research Letters, 14, p. 095005. doi.org/10.1088/1748-9326/ab28bb.
- Oliver, C. D., et al. (2014) Carbon, fossil fuel, and biodiversity mitigation with wood and forests. Journal of Sustainable Forestry, 33, pp. 248–275. doi.org/10.1080/10549811.2013.839386.
- Lippke, B. R., et al. (2004) CORRIM: Life-cycle environmental performance of renewable building materials. Forest Products Journal, 54, pp. 8–19.
- Field, J. L., et al. (2020) Robust paths to net greenhouse gas mitigation and negative emissions via advanced biofuels. Proceedings of the National Academy of Sciences of the United States of America, 117, pp. 21968–21977. doi.org/10.1073/pnas.1920877117.
- California forest carbon plan: Managing our forest landscapes in a changing climate. Available at: https://resources.ca.gov/CNRALegacyFiles/wp-content/uploads/2018/05/California-Forest-Carbon-Plan-Final-Draft-for-Public-Release-May-2018.pdf.
- Kalies, E. L., et al. (2016) Are fuel treatments effective at achieving ecological and social objectives? A systematic review. Forest Ecology and Management, 375, pp. 84–95. doi.org/10.1016/j.foreco.2016.05.021.
- Stephens, S. L., et al. (2020) Fire and climate change: Conserving seasonally dry forests is still possible. Frontiers in Ecology and the Environment. doi.org/10.1002/fee.2218.
- Agee, J. K., et al. (2005) Basic principles of forest fuel reduction treatments 21. Forest Ecology and Management, 1, pp. 83–96. doi.org/10.1016/j.foreco.2005.01.034.
- Gray, A. N., et al. (2016) Carbon stocks and accumulation rates in Pacific Northwest forests: Role of stand age, plant community, and productivity. Ecosphere, 7, p. e01224.
- Sanchez, D. L., et al. (2020) Literature Review and Evaluation of Research Gaps to Support Wood Products Innovation. Joint Institute for Wood Products Innovation.
- Lazarus, W. F., et al. (2011) Economic impacts of short-rotation woody crops for energy or oriented strand board: a Minnesota case study. 109, pp. 149–156.
- Meyer, P. A., et al. (2020) The effect of feedstock composition on fast pyrolysis and upgrading to transportation fuels: Techno-economic analysis and greenhouse gas life cycle analysis. Fuel, 259, p. 116218. doi.org/10.1016/j.fuel.2019.116218.
- Baker, S. E., et al. (2020) Getting to neutral: Options for negative carbon emissions in California. LLNL-TR-796100, Lawrence Livermore National Laboratory.
- Sierra Club California, Moving beyond incineration: Putting residues from California forest management and restoration to good use. Available at: https://www.sierraclub.org/sites/www.sierraclub.org/files/sce/sierra-club-california/PDFs/SCC_MovingBeyondIncineration.pdf.
- Smyth, C., et al. (2017) Estimating product and energy substitution benefits in national-scale mitigation analyses for Canada. Global Change Biology Bioenergy, 9, pp. 1071–1084. doi.org/10.1111/gcbb.12389.
- Geng, A., et al. (2019) Assessing the greenhouse gas mitigation potential of harvested wood products substitution in China. Environmental Science Technology, 53, pp. 1732–1740. doi.org/10.1021/acs.est.8b06510.
- North, M. P., et al. (2017) Cover of tall trees best predicts California spotted owl habitat. Forest Ecology Management, 405, pp. 166–178. doi.org/10.1016/j.foreco.2017.09.019.
- McWethy, D. B., et al. (2019) Rethinking resilience to wildfire. Nature Sustainability, 2, 797–804. doi.org/10.1038/s41893-019-0353-8.
- Millar, C. I., et al. (2007) Climate change and forests of the future: Managing in the face of uncertainty. Ecological Applications, 17, pp. 2145–2151. doi.org/10.1890/06-1715.1.
- California Assessment of Wood Business Innovation Opportunities and Markets. Available at: https://www.nationalforests.org/assets/pdfs/California-Assessment-Wood-Biomass-Innovation-Interim-Report-June-2015.pdf.
- BECK Group (2021) California Biomass Utilization Facility: Economic viability assessment. Available at: https://www.tuolumnecounty.ca.gov/.
- Finney, M. A., et al. (2011) A method for ensemble wildland fire simulation. Environment Modeling & Assessment. doi.org/10.1007/s10666-010-9241-3.
- Fried, J. S., et al. (2016) Inventory-based landscape-scale simulation of management effectiveness and economic feasibility with BioSum. Journal of Forestry. doi.org/10.5849/jof.15-087.
- Collins, B. M., et al. (2018) Fire weather and large fire potential in the northern Sierra Nevada. Agricultural and Forest Meteorology, 189–190, pp. 30–35. doi.org/10.1016/j.agrformet.2014.01.005.
- Collins, B. M., et al. (2019) A quantitative comparison of forest fires in central and northern California under early (1911-1924) and contemporary (2002-2015) fire suppression. International Journal of Wildland Fire.
- Campbell, J., et al. (2007) Law, Pyrogenic carbon emission from a large wildfire in Oregon, United States. Journal of Geophysical Research, 112, pp. 1–11. https://doi.org/10.1029/2007JG000451.
- Campbell, J. L., et al. (2016) Carbon emissions from decomposition of fire-killed trees following a large wildfire in Oregon, United States. Journal of Geophysical Research: Biogeosciences, 121, pp. 718–730. doi.org/10.1002/2015JG003165.
- Wang, M., et al. (2008) The Greenhouse Gases, Regulated Emissions, and Energy Use in Transportation (GREET) Model: Version 1.5. Center for Transportation Research, Argonne National Laboratory.
- US Environmental Protection Agency (2020) Data from “eGRID Summary Tables 2018.” Emissions & Generation Resource Integrated Database (eGRID). Available at: https://www.epa.gov/.
- Stewart, W. C., et al. (2012) Documenting the full climate benefits of harvested wood products in northern California: Linking harvests to the US greenhouse gas inventory. Forest Products Journal, 62, pp. 340–353. doi.org/10.13073/0015-7473-62.5.340.
- Skog, K. E., et al. (2008) Sequestration of carbon in harvested wood products for the United States. Forest Products Journal, 58, pp. 56–72.
- Wang, X., et al. (2011) Wood biodegradation in laboratory-scale landfills. Environmental Science Technology, 45, pp. 6864–6871. doi.org/10.1021/es201241g.
- Wang, X., et al. (2013) Barlaz, Decomposition of forest products buried in landfills. Waste Management, 33, pp. 2267–2276. doi.org/10.1016/j.wasman.2013.07.009.