Artificial light at night (ALAN) has a negative impact on natural systems worldwide. ALAN causes changes in physiology and behavior in organisms, which can have an impact on populations, communities, and ecosystems. ALAN’s confusing effect on nocturnal migration is one of the most serious consequences for birds.
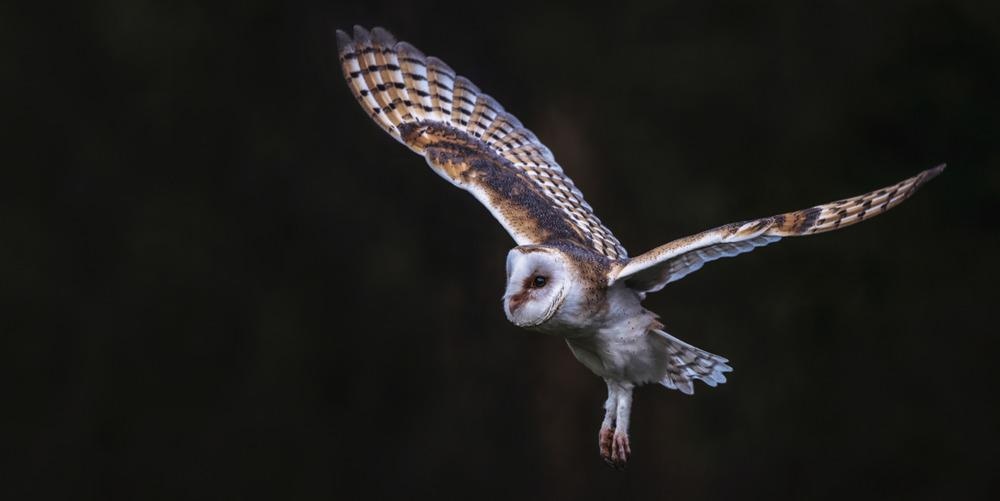
Image Credit: Albert Beukhof/Shutterstock.com
During the migratory flight, nocturnally migrating birds are drawn to ALAN on an individual level. During the stopover, populations of nocturnally migratory birds have been shown to be closer to ALAN, and species numbers have been demonstrated to be connected with ALAN sources in urban areas. This article will look at seasonal associations with light pollution trends and their effect on nocturnally migrating bird populations. The research was published in Ecosphere.
Outside of seasonal migration, ALAN can have a negative impact on nocturnally migratory birds. Urban sources of ALAN are related to decreased abundance and fewer nocturnally migratory species at the population level during both breeding and non-breeding seasons. ALAN may affect migratory and resident animals’ circadian rhythms, behavior, and physiology at the individual level.
ALAN is often treated as a static source of pollution in studies on the ecological impacts of ALAN on migrating birds. ALAN, on the other hand, is a dynamic phenomenon influenced by urban expansion and degradation, as well as technological advancements in lighting.
As a result, documenting the effects of ALAN requires using a whole annual cycle viewpoint. However, the spatial link between nocturnally migratory bird populations’ seasonal distributions and ALAN trends has not been investigated.
The goal of this research is to document how correlations with ALAN annual trends are defined across the entire annual cycle for nocturnally migrating birds, with the objective of enhancing baseline information on the regions and seasons where mitigation efforts like Lights Out programs would have the greatest impact.
Experts show how populations of nocturnally migratory bird species that nest in North America and travel throughout the Western Hemisphere are linked to ALAN patterns throughout the course of the year.
For the combined period 2005–2020, researchers compare weekly estimations of relative abundance for 42 nocturnally migrating passerine (NMP) bird species obtained from data from the eBird community science initiative with yearly estimates of ALAN for the period 1992–2013.
As a result, researchers anticipate that the 42 NMP species will be linked to favorable ALAN trends during the majority of their yearly life cycles.
Their goal is to inform ALAN mitigation efforts and increase the understanding of the ecological implications of various types of environmental pollution for birds and other species by validating these predictions.
Methodology
Researchers assessed the four seasons of the annual cycle (nonbreeding, spring migration, breeding, and fall migration) for the 42 NMP species using the following technique to support the interpretation seen in Figure 1.
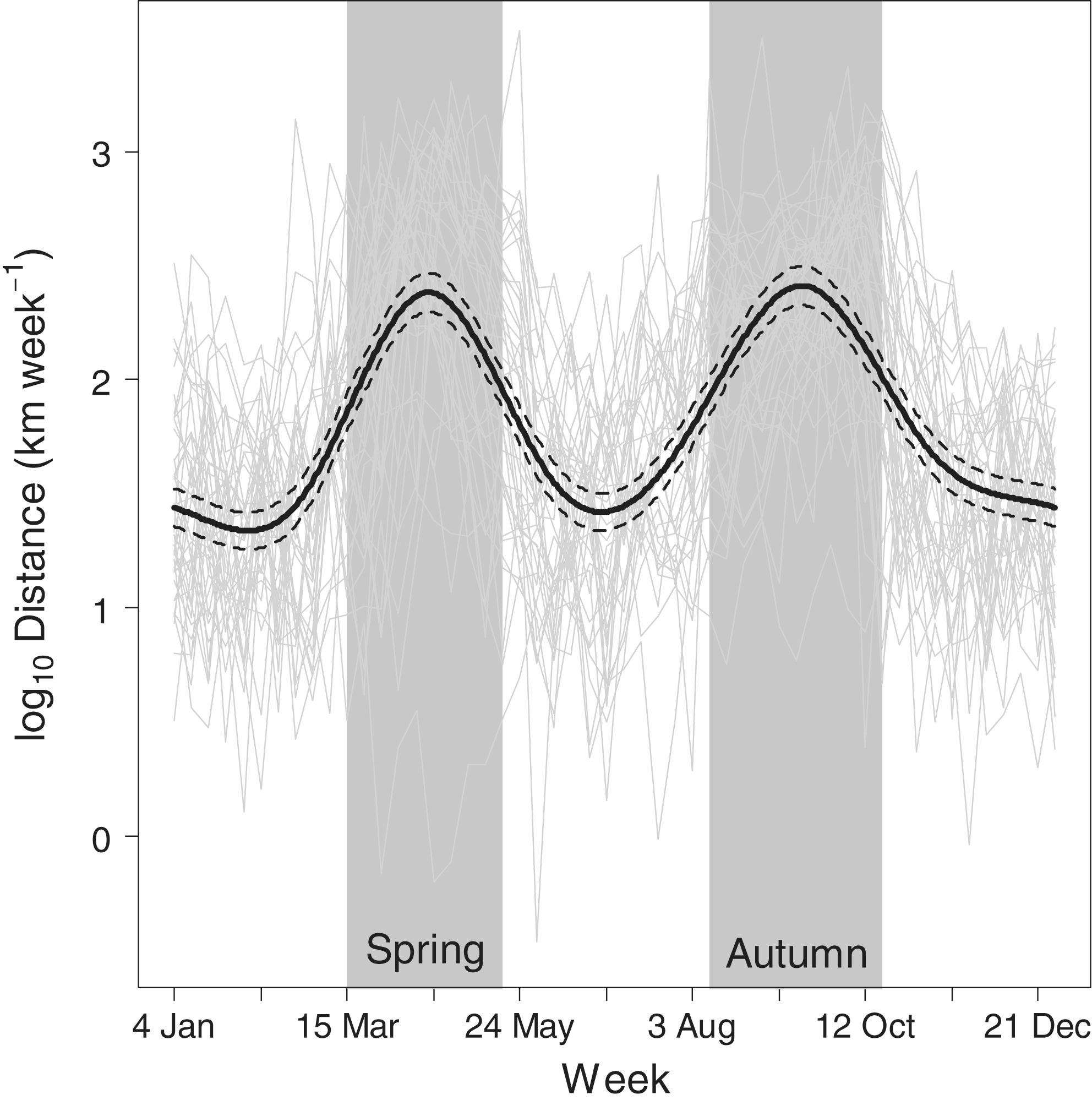
Figure 1. The great-circle (geodesic) distance between weekly centroids of occurrence weighted by relative abundance for 42 nocturnally migrating passerine bird species. The fitted black line and 95% confidence band are from a generalized additive mixed model (GAMM) with species included as a random effect. The vertical polygons demarcate spring migration (15 March–17 May) and autumn migration (10 August–19 October) as delineated by the inflection points in the fitted GAMM line. Image Credit: La Sorte, et al., 2022
Researchers estimated ALAN by year for the period 1992–2013 in the Western Hemisphere using the harmonized global nighttime light dataset normalized using stepwise calibration (see Figure 2).
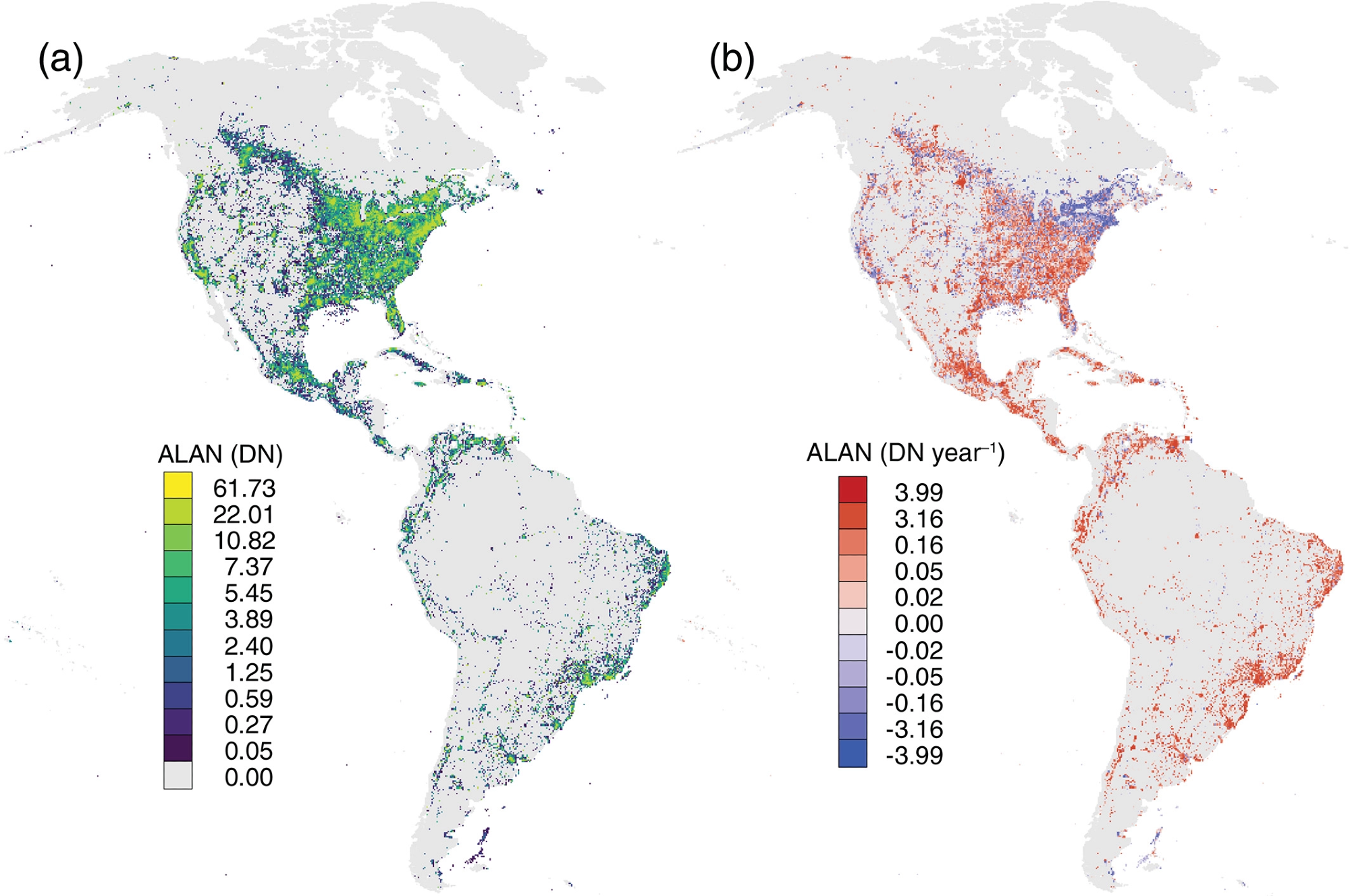
Figure 2. (a) Average artificial light at night (ALAN) and (b) the trend in ALAN during the period 1992–2013 within the Western Hemisphere. The ALAN data are gridded at a 30-arcsecond spatial resolution (ca. 1 km at the equator), and the units are digital numbers (DNs; range = 0–63). The trend analysis was implemented using ordinary least-squares regression. The data are displayed using a Mollweide equal-area projection. Image Credit: La Sorte, et al., 2022
Results
The 42 NMP species had different associations with ALAN annual trends depending on the week and the species, as depicted in Figure 3.
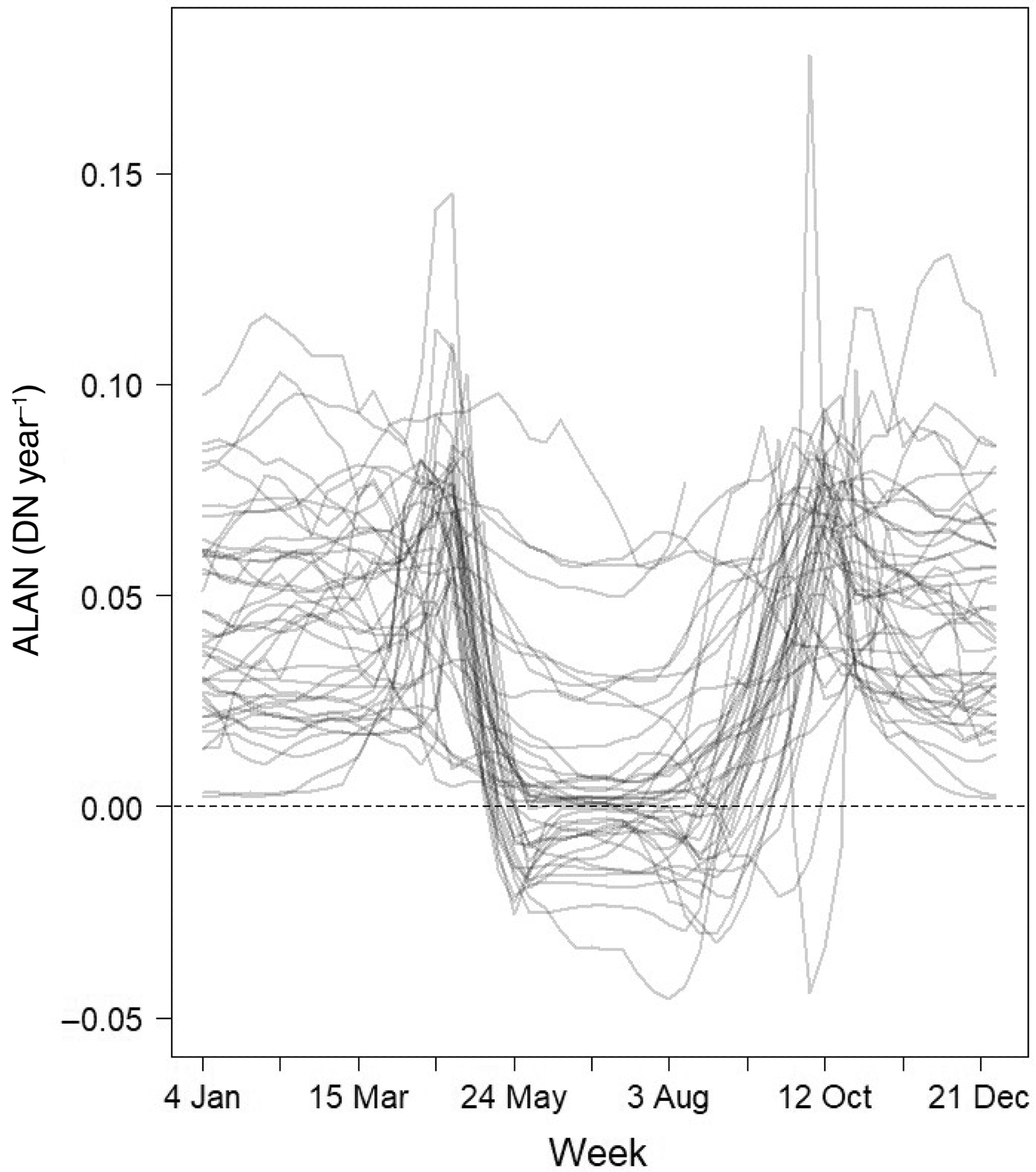
Figure 3. Weekly associations with trends in artificial light at night (ALAN) during the period 1992–2013 for 42 nocturnally migrating passerine bird species. Image Credit: La Sorte, et al., 2022
Figure 4 shows three significant clusters comprising 19, 15, and 8 species, respectively, discovered via hierarchical cluster analysis based on a minimum cluster size of eight species.
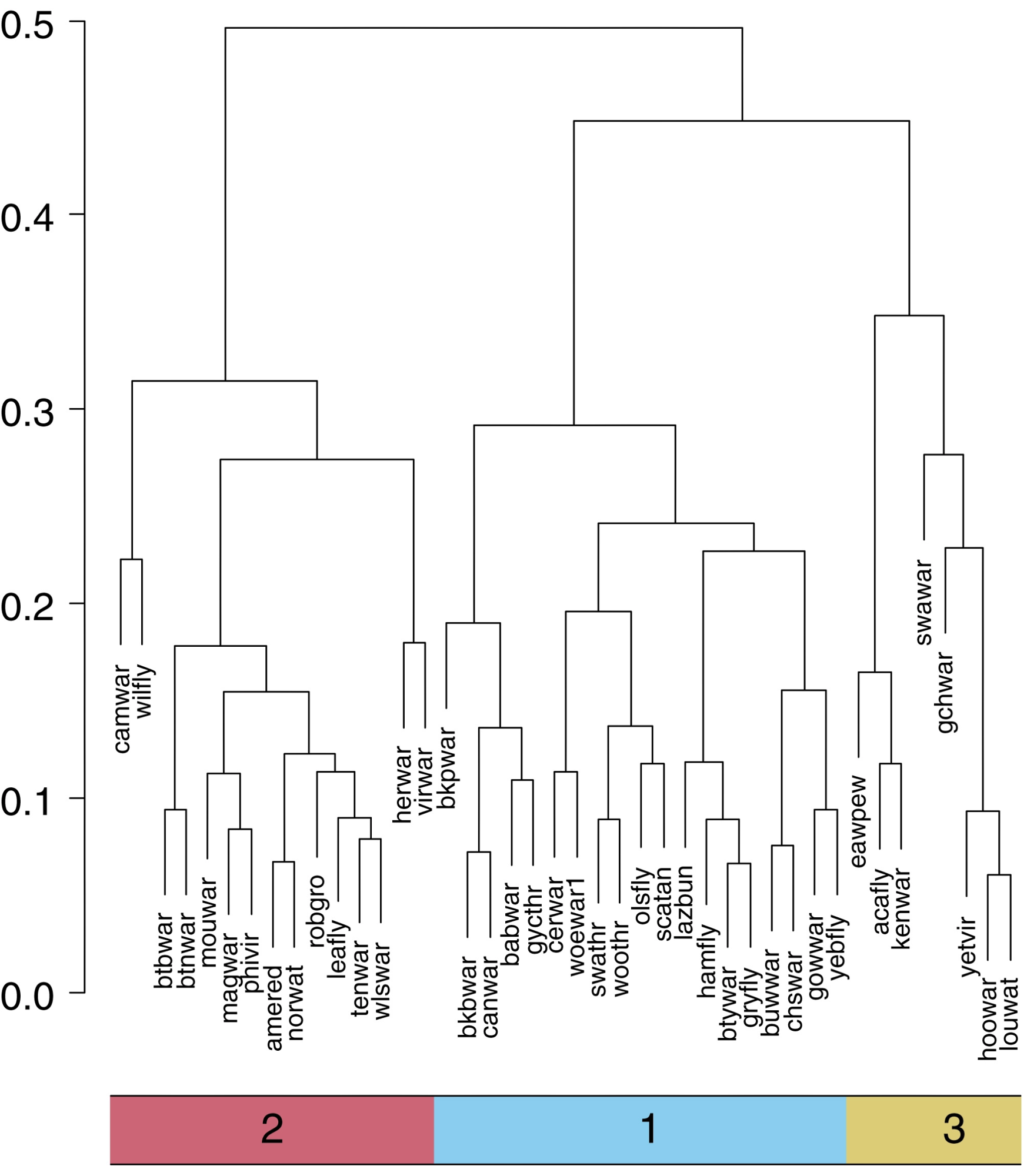
Figure 4. Dendrogram from a hierarchical cluster analysis of weekly associations with trends in artificial light at night for 42 nocturnally migrating passerine (NMP) bird species. The dendrogram labels are the common name alpha codes for the 42 NMP species. The colored annotations below the dendrogram identify species grouped into three clusters using an adaptive branch pruning technique. Image Credit: La Sorte, et al., 2022
In Figure 5, species in Clusters 1 and 3 were linked with low ALAN levels and positive ALAN trends during the non-breeding season, whereas species in Cluster 2 were associated with somewhat higher ALAN levels and greater positive ALAN trends.
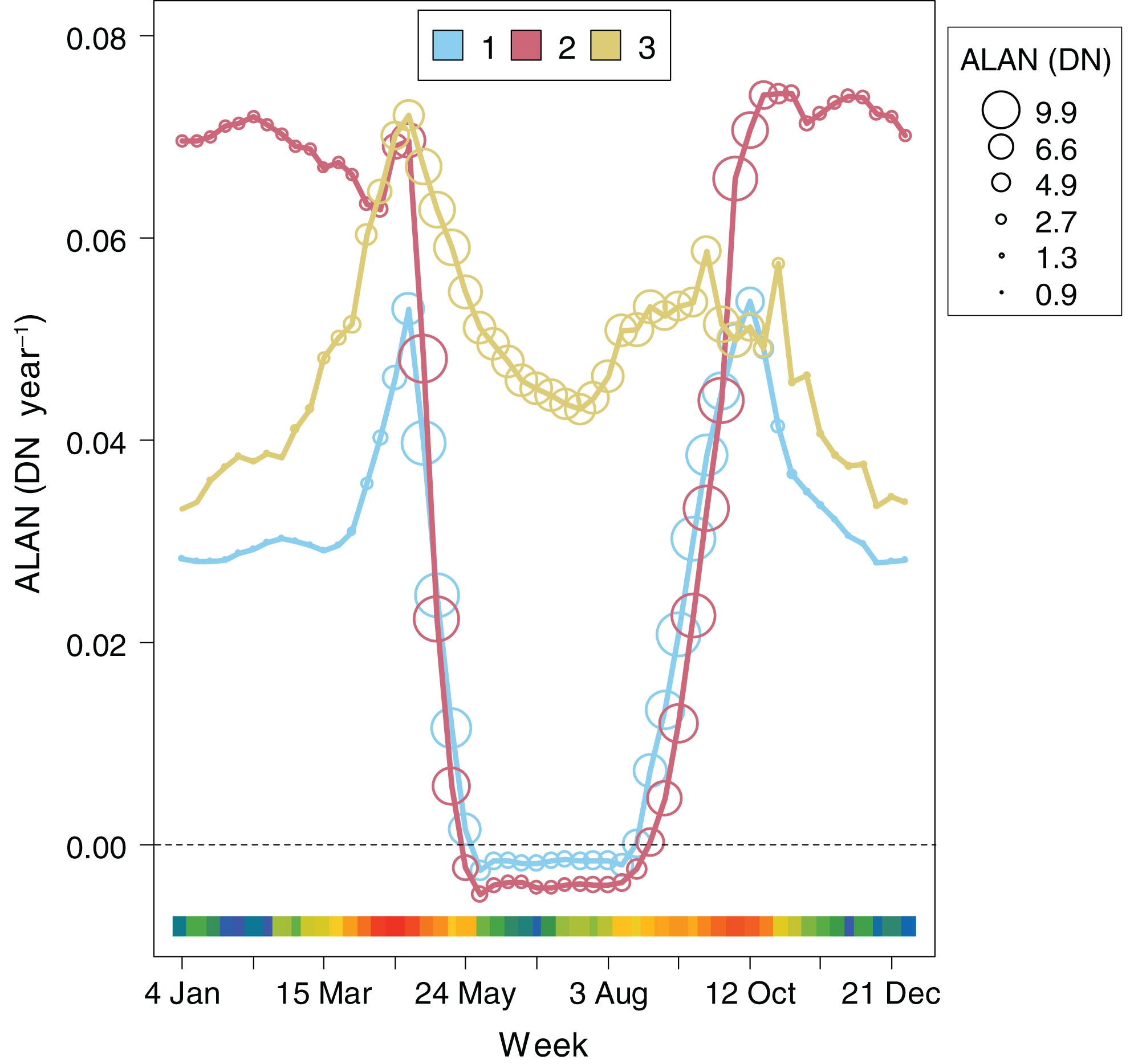
Figure 5. Weekly associations with trends in artificial light at night (ALAN) averaged across 42 nocturnally migrating passerine (NMP) bird species in three clusters (see Figure 4). The size of the circles corresponds to average ALAN. The sample sizes are 19, 15, and eight species, respectively. The color ramp is migration speed (see Figure 1) averaged across the 42 NMP species (blue = slow, green = intermediate, and red = fast). The ALAN units are digital numbers (DNs; range = 0–63). Image Credit: La Sorte, et al., 2022
Figure 6 shows the seasonal distributions of species in the three groups in the Western Hemisphere.
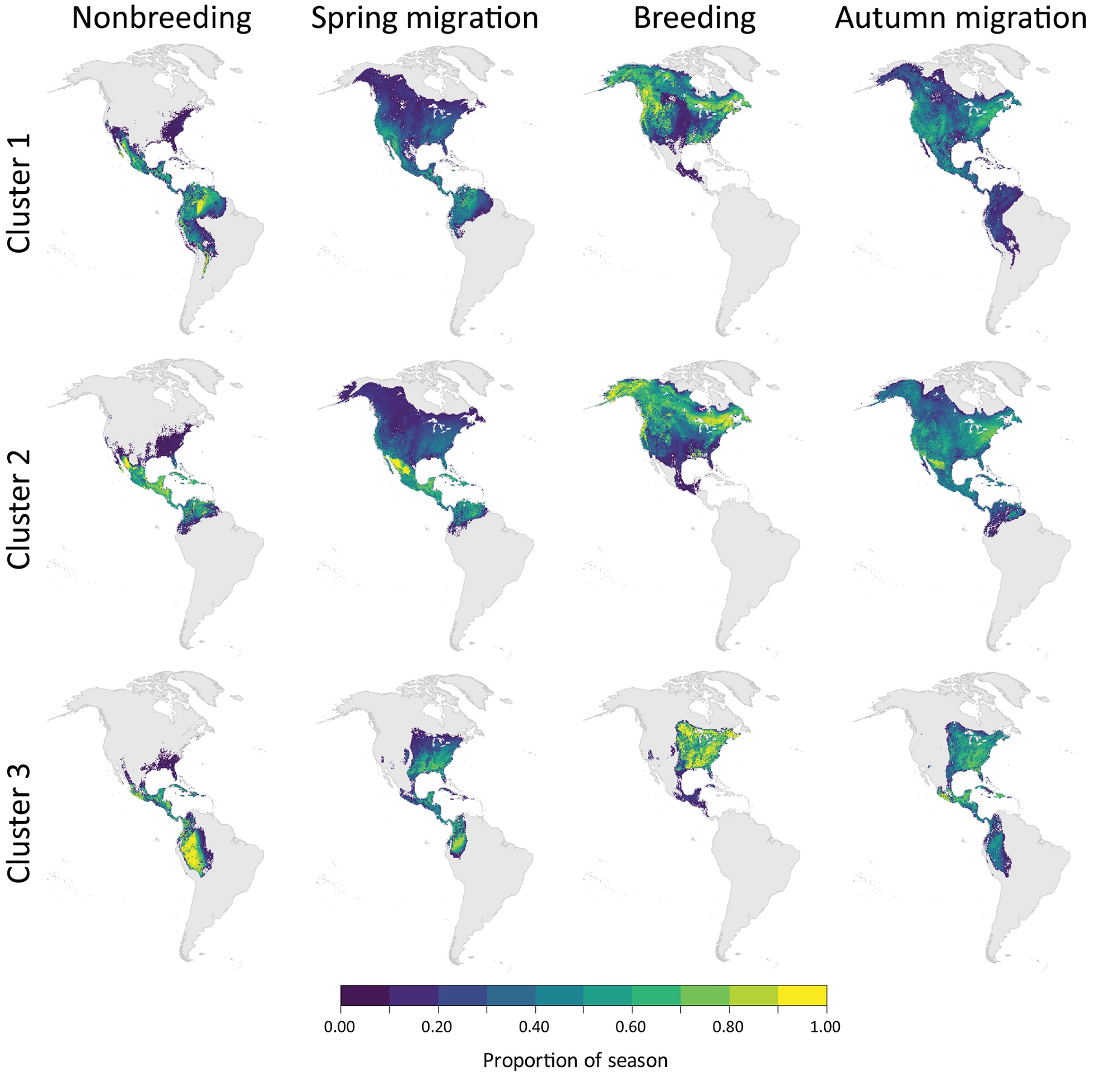
Figure 6. The seasonal distributions within the Western Hemisphere of 42 nocturnally migrating passerine bird species grouped into three clusters (n = 19, 15, and 8, respectively) based on their weekly associations with trends in artificial light at night (see Figure 3). The maps show the proportion of each season species occur in the grid cells averaged across species in each cluster. The data are displayed using a Mollweide equal-area projection. Image Credit: La Sorte, et al., 2022
Discussion
This research revealed three distinct clusters of NMP species, each of which had differing connections with ALAN trends based on weekly patterns of relative abundance in the Western Hemisphere. During the breeding season, two clusters of species were found in western and northern North America.
The species in these clusters had moderate levels of ALAN and somewhat negative ALAN trends. Species in these clusters were related to low ALAN levels and positive ALAN trends during the non-breeding season. Scientists discovered the third cluster of species whose positive ALAN trends persisted throughout the yearly cycle, peaking during migration, particularly in the spring.
During migratory and the non-breeding season, NMP species experience high ALAN levels and favorable ALAN trends in Central America, according to study findings. Central America’s unique topography necessitates large-scale migration methods inside the area.
Research data revealed that during the breeding season, southern North America had the greatest ALAN levels and strongest positive ALAN trends, whereas, during the non-breeding season, Central America had the highest ALAN levels and strongest positive ALAN trends.
Changes in lighting technology have traditionally influenced ALAN dynamics. This is now predicated on the shift to LED technology, which has resulted in increased ALAN emissions and changes in ALAN spectral composition in some areas. Depending on the scenario, switching to LED technology can either worsen or mitigate ALAN’s negative effects on birds.
It would be useful to analyze how LED technology, which has advanced since 2013, is affecting the ALAN patterns observed in this study, as well as the ramifications for the region’s nocturnally migratory bird species.
For many species, researchers summarized range-wide relationships with ALAN trends by week across the yearly cycle in this study. Exploring species-specific, local-scale connections with ALAN trends in data-poor locations, on the other hand, might be difficult. Efforts to improve the coverage of eBird data in under-sampled parts of the world might be beneficial in improving the spatial quality of these types of analyses.
Conclusion
Within the Western Hemisphere, research findings pinpoint the places and seasons when ALAN mitigation initiatives are most likely to provide the greatest benefits. These findings also lay the groundwork for further research into the impact of ALAN in recent bird population decreases in North America.
During migration, scientists identified Central America as a significant zone where reversing ALAN trends will likely benefit most individuals of the most species, particularly during spring migration.
Outside of migration, researchers found that reversing ALAN trends would likely have the greatest advantages in southern North America during the breeding season and Central America during the non-breeding season.
Because of urbanization and changes in lighting technology, the problems posed by ALAN for birds and other species will continue to develop, underlining the necessity of documenting ALAN relationships and their consequences at the individual and population levels across locations and seasons.
Journal Reference:
La Sorte, F.A., Horton, K.G., Johnston, A., Fink, D. and Auer, T. (2022) Seasonal associations with light pollution trends for nocturnally migrating bird populations. Ecosphere, 13(3), p.e3994. Available Online: https://esajournals.onlinelibrary.wiley.com/doi/10.1002/ecs2.3994.
References and Further Reading
- Amichai, E & N. Kronfeld-Schor (2019) “Artificial Light at Night Promotes Activity throughout the Night in Nesting Common Swifts (Apus apus).” Scientific Reports, 9, p. 11052. doi.org/10.1038/s41598-019-47544-3.
- Auer, T., et al. (2020) “ebirdst: Tools for Loading, Plotting, Mapping and Analysis of eBird Status and Trends Data Products.” R Package Version 0.2.0. https://cornelllabofornithology.github.io/ebirdst/.
- Aulsebrook, A. E., et al. (2020) “White and Amber Light at Night Disrupt Sleep Physiology in Birds.” Current Biology, 30, pp. 3657–63. doi.org/10.1016/j.cub.2020.06.085.
- Becker, D. J., et al. (2020) “Artificial Light at Night Amplifies Seasonal Relapse of Haemosporidian Parasites in a Widespread Songbird.” Proceedings of the Royal Society B: Biological Sciences, 287, p. 20201831. doi.org/10.1098/rspb.2020.1831.
- Bruderer, B., et al. (1999) “Behaviour of Migrating Birds Exposed to X-Band Radar and a Bright Light Beam.” Journal of Experimental Biology, 202, pp. 1015–22. doi.org/10.1242/jeb.202.9.1015.
- Cabrera-Cruz, S. A., et al. (2018) “Light Pollution Is Greatest within Migration Passage Areas for Nocturnally-Migrating Birds around the World.” Scientific Reports, 8, p. 3261. doi.org/10.1038/s41598-018-21577-6.
- de Jong, M., et al. (2017) “Early Birds by Light at Night: Effects of Light Color and Intensity on Daily Activity Patterns in Blue Tits.” Journal of Biological Rhythms, 32, pp. 323–33. doi.org/10.1177%2F0748730417719168.
- de Jong, M., et al. (2015) “Effects of Nocturnal Illumination on Life-History Decisions and Fitness in Two Wild Songbird Species.” Philosophical Transactions of the Royal Society B: Biological Sciences, 370, p. 20140128. doi.org/10.1098/rstb.2014.0128.
- Dominoni, D. M., et al. (2020) “Artificial Light at Night, in Interaction with Spring Temperature, Modulates Timing of Reproduction in a Passerine Bird.” Ecological Applications, 30, p. e02062. doi.org/10.1002/eap.2062.
- Falchi, F., et al. (2016) “The New World Atlas of Artificial Night Sky Brightness.” Science Advances, 2, p. e1600377. doi.org/10.1126/sciadv.1600377.
- Falcón, J., et al. (2020) “Exposure to Artificial Light at Night and the Consequences for Flora, Fauna, and Ecosystems.” Frontiers in Neuroscience, 14, p. 1183. doi.org/10.3389/fnins.2020.602796.
- Fink, D., et al. (2020) “Modeling Avian Full Annual Cycle Distribution and Population Trends with Citizen Science Data.” Ecological Applications, 30, p. e02056. doi.org/10.1002/eap.2056.
- Fink, D., et al. (2020) eBird Status and Trends, Data Version: 2018. Released: 2020. Ithaca, NY: Cornell Lab of Ornithology. doi.org/10.2173/ebirdst.2018.
- Fink, D., et al. (2013) “Adaptive Spatio-Temporal Exploratory Models: Hemisphere-Wide Species Distributions from Massively Crowdsourced eBird Data.” In Twenty-Seventh AAAI Conference on Artificial Intelligence (AAAI-13), Bellevue, WA.
- Gaston, K. J., et al. (2015) “The Biological Impacts of Artificial Light at Night: The Research Challenge.” Philosophical Transactions of the Royal Society B: Biological Sciences, 370, p. 20140133. doi.org/10.1098/rstb.2014.0133.
- Gauthreaux, S. A., et al. (2006) “Effects of Artificial Night Lighting on Migrating Birds.” In Ecological Consequences of Artificial Night Lighting, edited by C. Rich and T. Longcore, pp. 67–93. Washington, DC: Island Press.
- Hijmans, R. J. (2021) “geosphere: Spherical Trigonometry.” R Package Version 1. pp. 5–14. http://CRAN.R-project.org/package=geosphere.
- Horton, K. G., et al. (2019) “Bright Lights in the Big Cities: Migratory Birds' Exposure to Artificial Light.” Frontiers in Ecology and the Environment, 17, pp. 209–14. doi.org/10.1002/fee.2029.
- Hsu, F. C., et al. (2015) “DMSP-OLS Radiance Calibrated Nighttime Lights Time Series with Intercalibration.” Remote Sensing, 7, pp. 1855–76. doi.org/10.3390/rs70201855.
- Jiang, J., et al. (2020) “The Effects of Artificial Light at Night on Eurasian Tree Sparrow (Passer montanus): Behavioral Rhythm Disruption, Melatonin Suppression and Intestinal Microbiota Alterations.” Ecological Indicators, 108, p. 105702. doi.org/10.1016/j.ecolind.2019.105702.
- Kempenaers, B., et al. (2010) “Artificial Night Lighting Affects Dawn Song, Extra-Pair Siring Success, and Lay Date in Songbirds.” Current Biology, 20, pp. 1735–9. doi.org/10.1016/j.cub.2010.08.028.
- Kernbach, M. E., et al. (2021) “Light Pollution Affects West Nile Virus Exposure Risk across Florida.” Proceedings of the Royal Society B: Biological Sciences, 288, p. 20210253. doi.org/10.1098/rspb.2021.0253.
- Klaassen, R. H. G., et al. (2014) “When and where Does Mortality Occur in Migratory Birds? Direct Evidence from Long-Term Satellite Tracking of Raptors.” Journal of Animal Ecology, 83, pp. 176–84. doi.org/10.1111/1365-2656.12135.
- Kyba, C. C. M (2018) “Is Light Pollution Getting Better or Worse?” Nature Astronomy, 2, pp. 267–9. doi.org/10.1038/s41550-018-0402-7.
- Kyba, C. C. M., et al. (2017) “Artificially Lit Surface of Earth at Night Increasing in Radiance and Extent.” Science Advances, 3, p. e1701528. doi.org/10.1126/sciadv.1701528.
- La Sorte, F. A., et al. (2017) “Seasonal Associations with Urban Light Pollution for Nocturnally Migrating Bird Populations.” Global Change Biology, 23, pp. 4609–19. doi.org/10.1111/gcb.13792.
- La Sorte, F. A., et al. (2016) “Convergence of Broad-Scale Migration Strategies in Terrestrial Birds.” Proceedings of the Royal Society B: Biological Sciences, 283, p. 20152588. doi.org/10.1098/rspb.2015.2588.
- La Sorte, F A & K G Horton (2021) “Seasonal Variation in the Effects of Artificial Light at Night on the Occurrence of Nocturnally Migrating Birds in Urban Areas.” Environmental Pollution, 270, p. 116085. doi.org/10.1016/j.envpol.2020.116085.
- Langfelder, P., et al. (2008) “Defining Clusters from a Hierarchical Cluster Tree: The Dynamic Tree Cut Package for R.” Bioinformatics, 24, pp. 719–20. doi.org/10.1093/bioinformatics/btm563.
- Langfelder, P., et al. (2016) “dynamicTreeCut: Methods for Detection of Clusters in Hierarchical Clustering Dendrograms.” R Package Version 1.63–1. https://CRAN.R-project.org/package=dynamicTreeCut.
- Lao, S., et al. (2020) “The Influence of Artificial Light at Night and Polarized Light on Bird-Building Collisions.” Biological Conservation, 241, p. 108358. doi.org/10.1016/j.biocon.2019.108358.
- Larkin, R P & B A Frase (1988) “Circular Paths of Birds Flying near a Broadcasting Tower in Cloud.” Journal of Comparative Psychology, 102, p. 90–3. doi.apa.org/doi/10.1037/0735-7036.102.1.90.
- Li, X & Y Zhou (2017a) “A Stepwise Calibration of Global DMSP/OLS Stable Nighttime Light Data (1992–2013).” Remote Sensing, 9, p. 637. doi.org/10.3390/rs9060637.
- Li, X & Y Zhou (2017b) “Urban Mapping Using DMSP/OLS Stable Night-Time Light: A Review.” International Journal of Remote Sensing, 38, pp. 6030–46. doi.org/10.1080/01431161.2016.1274451.
- Li, X., et al. (2020) “A Harmonized Global Nighttime Light Dataset 1992–2018.” Scientific Data, 7, p. 168. doi.org/10.1038/s41597-020-0510-y.
- Lok, T., et al. (2015) “The Cost of Migration: Spoonbills Suffer Higher Mortality during Trans-Saharan Spring Migrations Only.” Biology Letters, 11, p. 20140944. doi.org/10.1098/rsbl.2014.0944.
- Loonstra, A. H. J., et al. (2019) “Adverse Wind Conditions during Northward Sahara Crossings Increase the in-Flight Mortality of Black-Tailed Godwits.” Ecology Letters, 22, pp. 2060–6. doi.org/10.1111/ele.13387.
- Loss, S. R., et al. (2014) “Bird–Building Collisions in the United States: Estimates of Annual Mortality and Species Vulnerability.” The Condor, 116, pp. 8–23. doi.org/10.1650/CONDOR-13-090.1.
- Malek, I & A Haim (2019) “Bright Artificial Light at Night Is Associated with Increased Body Mass, Poor Reproductive Success and Compromised Disease Tolerance in Australian Budgerigars (Melopsittacus undulatus).” Integrative Zoology, 14, pp. 589–603. doi.org/10.1111/1749-4877.12409.
- Marra, P. P., et al. (2015) “A Call for Full Annual Cycle Research in Animal Ecology.” Biology Letters, 11, p. 20150552. doi.org/10.1098/rsbl.2015.0552.
- McLaren, J. D., et al. (2018) “Artificial Light at Night Confounds Broad-Scale Habitat Use by Migrating Birds.” Ecology Letters, 21, pp. 356–64. doi.org/10.1111/ele.12902.
- Miller, S. D., et al. (2012) “Suomi Satellite Brings to Light a Unique Frontier of Nighttime Environmental Sensing Capabilities.” Proceedings of the National Academy of Sciences of the United States of America, 109, pp. 15706–11.
- Miller, S. D., et al. (2013) “Illuminating the Capabilities of the Suomi National Polar-Orbiting Partnership (NPP) Visible Infrared Imaging Radiometer Suite (VIIRS) Day/Night Band.” Remote Sensing, 5, pp. 6717–66. doi.org/10.3390/rs5126717.
- Nair, G. B & S. J. Dhoble (2015) “A Perspective Perception on the Applications of Light-Emitting Diodes.” Luminescence, 30, pp. 1167–75. doi.org/10.1002/bio.2919.
- Poot, H., et al. (2008) “Green Light for Nocturnally Migrating Birds.” Ecology and Society, 13, p. 47.
- R Development Core Team (2021) R: A Language and Environment for Statistical Computing. Vienna: R Foundation for Statistical Computing. www.R-project.org/.
- Rebke, M., et al. (2019) “Attraction of Nocturnally Migrating Birds to Artificial Light: The Influence of Colour, Intensity and Blinking Mode under Different Cloud Cover Conditions.” Biological Conservation, 233, pp. 220–7. doi.org/10.1016/j.biocon.2019.02.029.
- Rich, C & T Longcore (2013) Ecological Consequences of Artificial Night Lighting. Washington, DC: Island Press.
- Ridgely, R. S., et al. (2007) Digital Distribution Maps of the Birds of the Western Hemisphere, Version 3.0. Arlington, VA: NatureServe.
- Rosenberg, K. V., et al. (2019) “Decline of the North American Avifauna.” Science, 366, pp. 120–4. doi.org/10.1126/science.aaw1313.
- Sanders, D., et al. (2020) “A Meta-Analysis of Biological Impacts of Artificial Light at Night.” Nature Ecology & Evolution, 5, pp. 74–81. doi.org/10.1038/s41559-020-01322-x.
- Sanders, D & K. J. Gaston (2018) “How Ecological Communities Respond to Artificial Light at Night.” Journal of Experimental Zoology Part A: Ecological and Integrative Physiology, 329, pp. 394–400. doi.org/10.1002/jez.2157.
- Sergio, F., et al. (2019) “When and Where Mortality Occurs throughout the Annual Cycle Changes with Age in a Migratory Bird: Individual vs Population Implications.” Scientific Reports, 9, p. 17352. doi.org/10.1038/s41598-019-54026-z.
- Shimodaira, H (2002) “An Approximately Unbiased Test of Phylogenetic Tree Selection.” Systematic Biology, 51, pp. 492–508. doi.org/10.1080/10635150290069913.
- Shimodaira, H (2004) “Approximately Unbiased Tests of Regions Using Multistep-Multiscale Bootstrap Resampling.” Annals of Statistics, 32, pp. 2616–41. doi.org/10.1214/009053604000000823.
- Smith, R. A., et al. (2021) “Pre-Migration Artificial Light at Night Advances the Spring Migration Timing of a Trans-Hemispheric Migratory Songbird.” Environmental Pollution, 269, p. 116136. doi.org/10.1016/j.envpol.2020.116136.
- Sullivan, B. L., et al. (2014) “The eBird Enterprise: An Integrated Approach to Development and Application of Citizen Science.” Biological Conservation, 169, pp. 31–40. doi.org/10.1016/j.biocon.2013.11.003.
- Sun, J., et al. (2017) “Artificial Light at Night Affects Sleep Behaviour Differently in Two Closely Related Songbird Species.” Environmental Pollution, 231, pp. 882–9. doi.org/10.1016/j.envpol.2017.08.098.
- Suzuki, R.., et al. (2019) “pvclust: Hierarchical Clustering with P-Values via Multiscale Bootstrap Resampling.” R Package Version 2. pp. 2–0. https://CRAN.R-project.org/package=pvclust.
- United Nations. (2019) World Urbanization Prospects: The 2018 Revision (ST/ESA/SER.A/420). New York: Department of Economic and Social Affairs, Population Division, United Nations.
- Van Doren, B. M., et al. (2017) “High-Intensity Urban Light Installation Dramatically Alters Nocturnal Bird Migration.” Proceedings of the National Academy of Sciences of the United States of America, 114, pp. 11175–80. doi.org/10.1073/pnas.1708574114.
- Van Doren, B. M., et al. (2021) “Drivers of Fatal Bird Collisions in an Urban Center.” Proceedings of the National Academy of Sciences of the United States of America, 118, p. e2101666118. doi.org/10.1073/pnas.2101666118.
- Varadhan, R (2015) “features: Feature Extraction for Discretely-Sampled Functional Data.” Johns Hopkins University and MKG Subramaniam and AT&T Research Labs. R Package Version 2015. pp. 12–1. https://CRAN.R-project.org/package=features.
- Welbers, A. A. M. H., et al. (2017) “Artificial Light at Night Reduces Daily Energy Expenditure in Breeding Great Tits (Parus major).” Frontiers in Ecology and Evolution, 5, p. 55. doi.org/10.3389/fevo.2017.00055.
- Wood, S. N (2017) Generalized Additive Models: An Introduction with R, 2nd ed. Boca Raton, FL: Chapman & Hall/CRC. doi.org/10.1201/9781315370279.
- Wood, S. N & F. Scheipl (2020) “gamm4: Generalized Additive Mixed Models Using Mgcv and lme4.” R Package Version 0. pp. 2–6. https://CRAN.R-project.org/package=gamm4.
- Zhao, X., et al. (2020) “Blue Light Attracts Nocturnally Migrating Birds.” The Condor, 122, pp. 1–12. doi.org/10.1093/condor/duaa002.
- Zurell, D., et al. (2018) “Long-Distance Migratory Birds Threatened by Multiple Independent Risks from Global Change.” Nature Climate Change, 8, pp. 992–6. doi.org/10.1038/s41558-018-0312-9.