Cities are the epicenters of anthropogenic environmental inputs worldwide. Urban runoff, in particular, is a major source of pollution that contaminates aquatic environments. As the size of cities (which are mostly made up of impermeable surfaces such as asphalt and concrete) grows, so does urban runoff, which has a direct influence on surface water quality and storage. This article looks at a study on sustainable strategies to treat urban runoff published in Nature Sustainability.
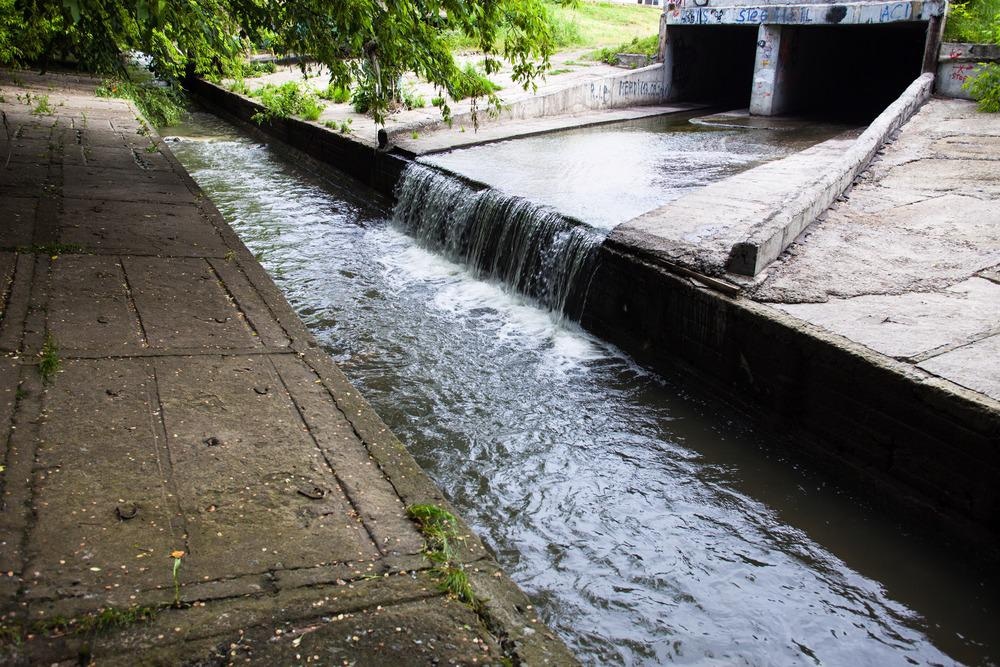
Image Credit: rootstock/Shutterstock.com
Plastic debris, detergents, hydrocarbons, solvents, viruses, pesticides, heavy metals, and engineered nanomaterials are among the hazardous, non-biodegradable, and emerging pollutants released into the environment by urban runoff in general.
The condition known as urban runoff mortality syndrome explains enormous salmon deaths caused by untreated stormwater. Pollutant emissions might be controlled by international actions and policies, limiting negative ecological effects and long-distance transfer.
Researchers assume that cities, especially those encircled by a small hydrographic system where pollutant dilution is low after outflow into natural waters, should recognize assigning more resources to characterize and treat urban runoff, similar to how industrial or municipally treated wastewaters are treated.
Although stormwater best management practices (BMPs) are in place in some big cities, they are primarily designed for water infiltration and storage, as well as to deal with heavy rainfall events. They do not address anthropogenic stresses in the area.
With increased concerns about floods linked to global climate change, a stormwater treatment system’s local flow-buffering capability is crucial. Urban planners and water managers, on the other hand, lack dependable methods for designing urban stormwater management infrastructure that considers both flood and water-quality control.
Researchers emphasize the necessity for routine characterization of urban runoff as well as new infrastructure and instrumentation investments intended to improve stormwater management in the long run.
Methodology
The cocktail of pollutants dumped into natural waterways by urban runoff (~110 billion gallons per year in New York City alone) goes beyond the traditional water-quality indicators (for example, phosphorus, total suspended solids, metals, and nitrogen).
Every day, plenty of additional pollutants are discharged into natural waters via urban stormwater runoff and stormwater drainage systems, some of which are hazardous to aquatic creatures. This is shown in Figure 1.
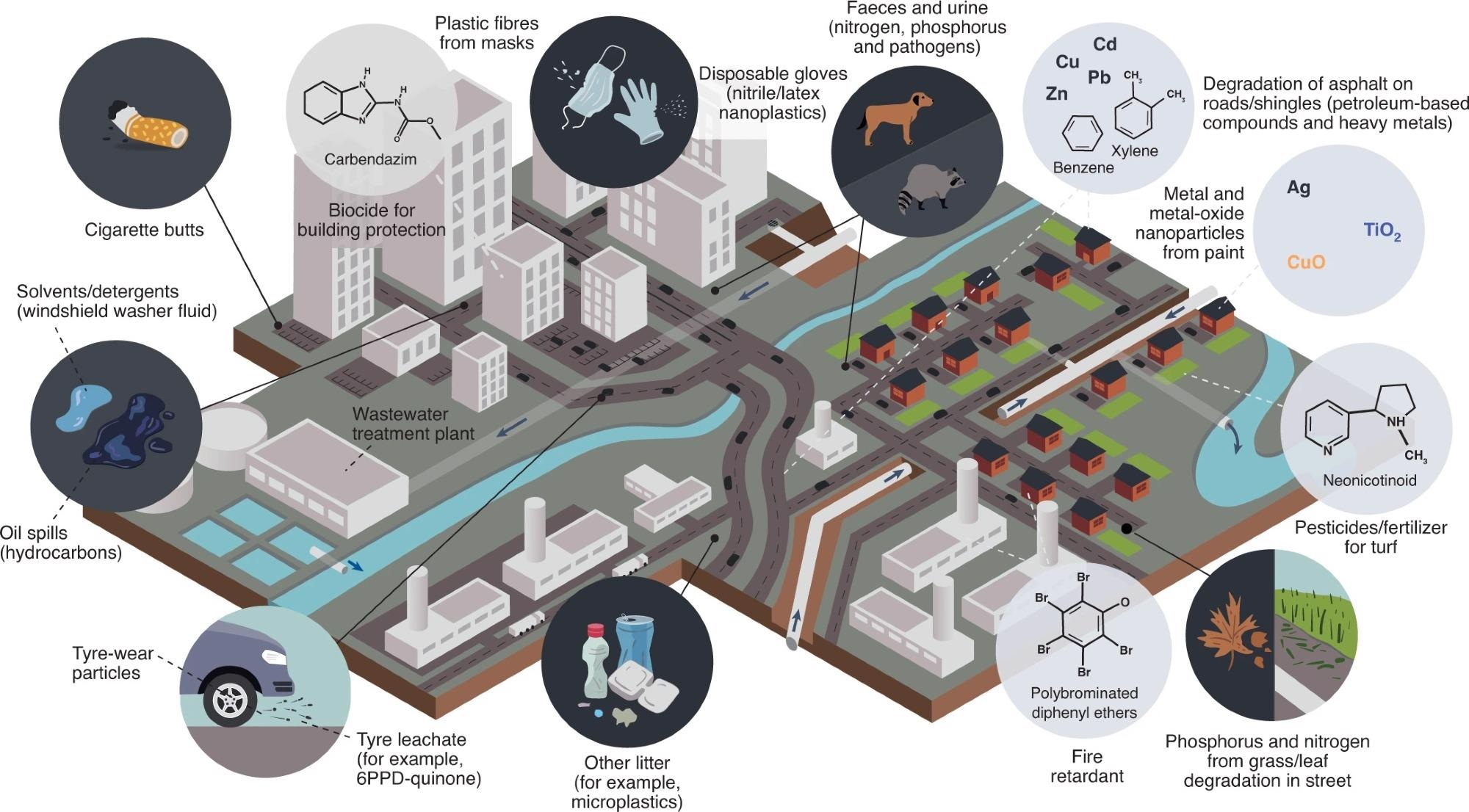
Figure 1. Mapping global anthropogenic pressures from conventional and emerging contaminants. Runoffs are not treated. Image Credit: Lapointe, et al., 2022
Other plastic-based litter, in addition to tire-wear debris, is widespread in stormwater runoff. This load is additionally enhanced by combined sewer overflows transporting untreated municipal wastewaters. Furthermore, the widespread usage of single-use personal protective equipment (estimated at ~15, 50 billion masks and 780 billion gloves yearly) as a result of the SARS-CoV-2 epidemic leads to the emission of plastic litter.
Gasoline and oil spills, heavy metals, and petroleum-based substances (for example, polycyclic aromatic hydrocarbons (PAHs)) from asphalt-based surfaces such as rooftops and roadways can cause concern. A Colombian river had nearly 4,500 ng PAH l–1, even though several PAHs have a median lethal value (LC50) of less than 2,000 ng l–1. Finally, engineered nanoparticles can be liberated from paints and stains used on outdoor surfaces.
Many additional anthropogenic pollutants, including salts and de-icing chemicals used in the winter, detergents, solvents (for example, windshield washers), and brominated flame retardants, are discharged into natural waterways following significant precipitation events.
Others remain unidentified. Furthermore, high numbers of city-acclimated and domestic animals (such as skunks, mustelids, birds, raccoons, squirrels, and cats) may contribute to infections, organic phosphorus, and nitrogen (urea) discharge in natural streams.
Importantly, the amounts of these pollutants are not routinely tested, and their cumulative toxicity effects are unknown and perhaps overestimated. To assess the danger to aquatic ecosystems and identify places where mitigation techniques are needed, a deeper knowledge of the loadings of pollutants and any related toxicity is required.
Simple indicators such as turbidity may be useful in estimating the load of colloidal pollutants, and new inventive low-cost technologies are essential to monitoring target contaminants (for example, microplastics).
Furthermore, BMPs can be implemented to reduce pollution. To better assess risk, strategically located dual systems that can statistically and qualitatively monitor runoff might become increasingly widespread in cities (in bigger sewers). Governments and scientists must conduct monitoring to determine whether more active management is required and whether urban runoff should be processed before being discharged into the environment.
Discussion
During peak rainfall or snowmelt events, new and strategically geolocated infiltration zones, collecting systems, and/or modular treatment procedures that give some expansion flexibility can assist decrease floods and a load of pollutants.
To collect and passively treat urban runoff, as well as deal with strong rainstorm events that cannot be physically sustained by conventional wastewater treatment plants built to handle lower flow rates, large-scale feasible and sustainable solutions are required.
Figure 2 shows examples of existing and new promising solutions.
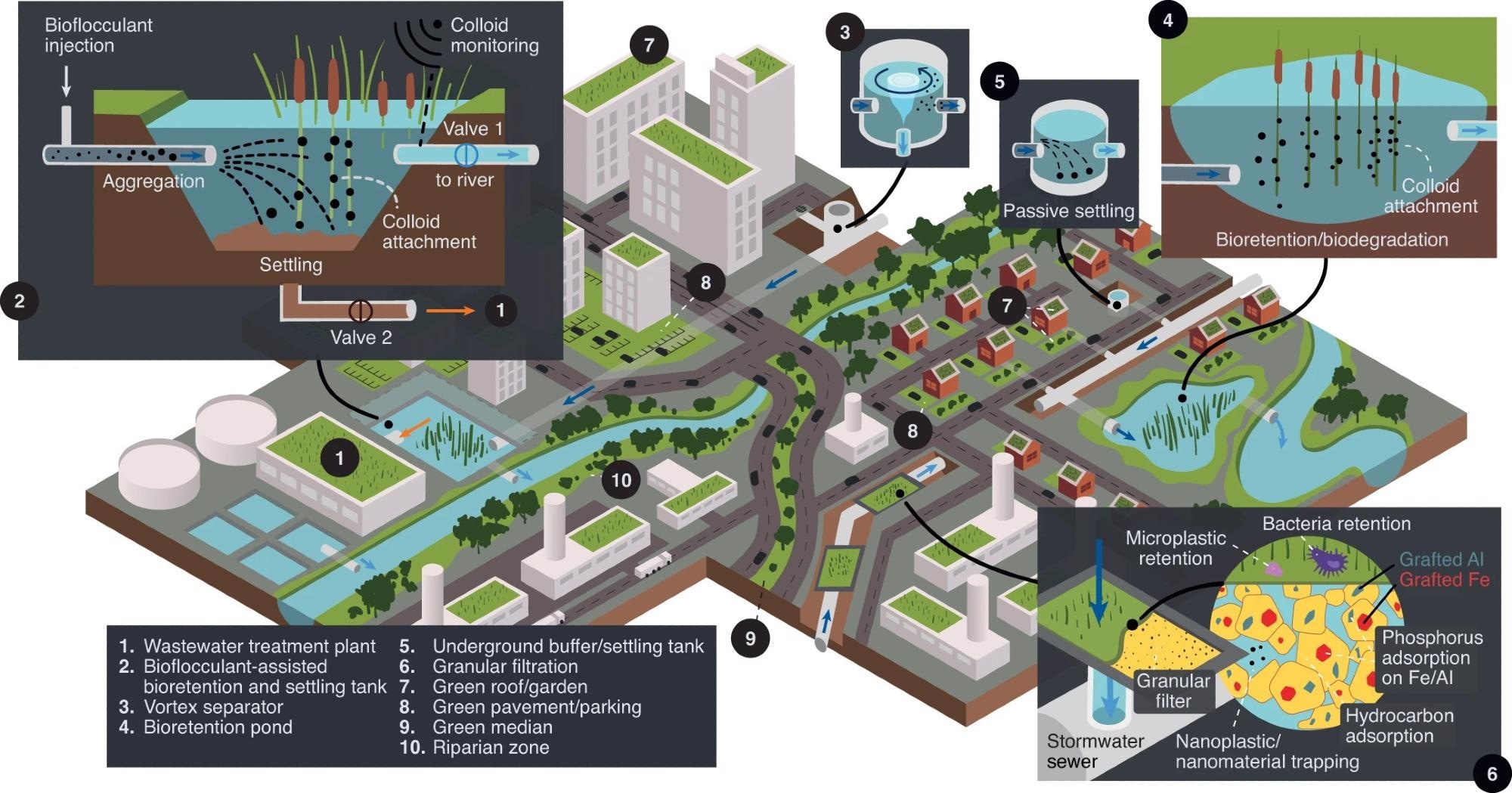
Figure 2. Solutions to treat runoff. Each number represents a different solution for runoff treatment, as shown in the corresponding key. In solution 2, contaminant aggregation or settling is improved with (bio) flocculants. Valve 2 is open to send settled sludge to 1 and clean water is sent back to the river. Valve 1 is open when turbidity is acceptable, or closed for longer settling. In solutions 3 and 5, centripetal and gravitational forces, respectively, separate large/dense solids from water. In solution 4, no chemical is added, colloids are removed via passive settling and bioretention. Longer residence times enable biofilm formation and biodegradation. In solution 6, recycled crushed glass grafted with metals improves colloid retention and adsorption of soluble contaminants via electrostatic interactions. In solutions 7–10, colloids and soluble contaminants are removed and the processes also act as hydraulic buffers. Arrows in dark and light blue indicate raw and treated waters, respectively. Image Credit: Lapointe, et al., 2022
Furthermore, certain systems might be built to deal with the “first flush” of runoff to minimize costs and improve the integration of such solutions in congested cities, as the initial rainfall normally releases larger pollutant loads.
The quantity of green space in dense urban areas has been linked to human health and economical advantages, in addition to improving water quality and helping to conserve biodiversity and offset urban heat island impacts.
In the United States, green treatment infrastructure accounts for <10% (US$4.2 billion) of the total capital investments (US$48.0 billion) made to combat combined sewer overflows and achieve the Clean Water Act’s water-quality goals.
Green infrastructures that are now in place are geolocated and intended to manage floods and water buildup. If cities want to build more adaptable green infrastructure, they must address both water storage and treatment needs.
Furthermore, local pollution patterns and water properties will have a big impact on the sort of technology used (granular filter vs. adsorbent, for example). For instance, runoff containing large quantities of suspended solids (such as sand and tire-wear particles) may necessitate different methods than runoff containing high levels of soluble phosphorus.
Conclusion
Cities have a certain amount of resources for stormwater management. As a result, the proposed methods might be combined with more advanced process control or data-driven machine-learning approaches to maximize the cost-effectiveness of present and future green infrastructures, as well as to lower the risk of acute toxicity in natural waterways.
The data stream might comprise rainfall intensity–duration–frequency curves, stormwater models, weather predictions, unexpected and planned events (for example, hydrocarbon spills and salts applied in the winter), unique qualitative and quantitative tools, and river flows.
Because few rules limit the design of solutions, towns should have some flexibility in implementing locally tailored, practical, sustainable, and low-cost procedures. Despite the difficulty of implementing new runoff processes, researchers suggest that cities should examine such comprehensive solutions when chances for infrastructure modifications occur.
This might help to reduce and prevent toxic runoff from entering aquatic ecosystems, protecting animals, people, and resources that are vital to the global communities.
Journal Reference
Lapointe, M., Rochman, C.M. and Tufenkji, N. (2022) Sustainable strategies to treat urban runoff needed. Nature Sustainability, pp.1-4. Available Online: https://www.nature.com/articles/s41893-022-00853-4.
References and Further References
- Cooley, S. W., et al. (2021) Human alteration of global surface water storage variability. Nature, 591, pp. 78–81. doi.org/10.1038/s41586-021-03262-3.
- Tian, Z., et al. (2021) A ubiquitous tire rubber–derived chemical induces acute mortality in coho salmon. Science, 371, pp. 185–189. doi.org/10.1126/science.abd6951.
- Chow, M. I., et al. (2019) An urban stormwater runoff mortality syndrome in juvenile coho salmon. Aquatic Toxicology, 214, p. 105231. doi.org/10.1016/j.aquatox.2019.105231.
- Wang, Z., et al. (2021) We need a global science-policy body on chemicals and waste. Science, 371, pp. 774–776. doi.org/10.1126/science.abe9090.
- Hopkins, K. G., et al. (2018) Influence of governance structure on green stormwater infrastructure investment. Environmental Science & Policy, 84, pp. 124–133. doi.org/10.1016/j.envsci.2018.03.008.
- Zhu, X., et al. (2021) Holistic Assessment of Microplastics and Other Anthropogenic Microdebris in an Urban Bay Sheds Light on Their Sources and Fate. ES&T Water, 1, pp. 1401–1410. doi.org/10.1002/sus2.1.
- Prata, J. C., et al. (2020) COVID-19 Pandemic Repercussions on the Use and Management of Plastics. Environmental Science & Technology, 54, pp. 7760–7765. doi.org/10.1021/acs.est.0c02178.
- Araújo, M C B & Costa, M F (2019) A critical review of the issue of cigarette butt pollution in coastal environments. Environmental Research, 172, pp. 137–149. doi.org/10.1016/j.envres.2019.02.005.
- Sarria-Villa, R., et al. (2016) Presence of PAHs in water and sediments of the Colombian Cauca River during heavy rain episodes, and implications for risk assessment. Science of the Total Environment, 540, pp. 455–465. doi.org/10.1016/j.scitotenv.2015.07.020.
- Newsted, J L & Giesy, J P (1987) Predictive models for photoinduced acute toxicity of polycyclic aromatic hydrocarbons to Daphnia magna, strauss (cladocera, crustacea). Environmental Toxicology and Chemistry, 6, pp. 445–461. doi.org/10.1002/etc.5620060605.
- Bollmann, U. E., et al. (2014) Dynamics of biocide emissions from buildings in a suburban stormwater catchment – Concentrations, mass loads and emission processes. Water Research, 56, pp. 66–76. doi.org/10.1016/j.watres.2014.02.033.
- Pike, K. A., et al. (2021) Correlation Analysis of Perfluoroalkyl Substances in Regional U.S. Precipitation Events. Water Research, 190, p. 116685. doi.org/10.1016/j.watres.2020.116685.
- Lapointe, M., et al. (2020) Understanding and Improving Microplastic Removal during Water Treatment: Impact of Coagulation and Flocculation. Environmental Science & Technology, 54, pp. 8719–8727. doi.org/10.1021/acs.est.0c00712.
- Mentens, J., et al. (2006) Green roofs as a tool for solving the rainwater runoff problem in the urbanized 21st century?. Landscape and Urban Planning, 77, pp. 217–226. doi.org/10.1016/j.landurbplan.2005.02.010.
- O’Regan, A. C., et al. (2021) “Biophilic Cities”: Quantifying the Impact of Google Street View-Derived Greenspace Exposures on Socioeconomic Factors and Self-Reported Health. Environmental Science & Technology, 55, pp. 9063–9073. doi.org/10.1021/acs.est.1c01326.
- Wong, N. H., et al. (2003) Life cycle cost analysis of rooftop gardens in Singapore. Building and Environment, 38, pp. 499–509. doi.org/10.1016/S0360-1323(02)00131-2.
- Fitzgerald, J & Laufer, J (2017) Governing green stormwater infrastructure: the Philadelphia experience. Local Environment: The International Journal of Justice and Sustainability, 22, pp. 256–268. doi.org/10.1080/13549839.2016.1191063.
- Clean Watersheds Needs Survey 2012: Report to Congress EPA-830-R-15005 (US EPA, 2016)
- Li, C., et al. (2021) Evaluating the runoff storage supply-demand structure of green infrastructure for urban flood management. Journal of Cleaner Production, 280, p. 124420. doi.org/10.1016/j.jclepro.2020.124420.
- Hino, M., et al. (2018) Machine learning for environmental monitoring. Nature Sustainability, 1, pp. 583–588. doi.org/10.1038/s41893-018-0142-9.