Over half the energy fed into industrial processes is lost as waste heat; it simply vanishes into the surrounding environment as a ubiquitous form of thermal energy. This heat loss is inevitable, resulting in increased fuel usage to achieve the desired energy output. As a consequence, a rise in greenhouse gas emissions and global warming is unavoidable.
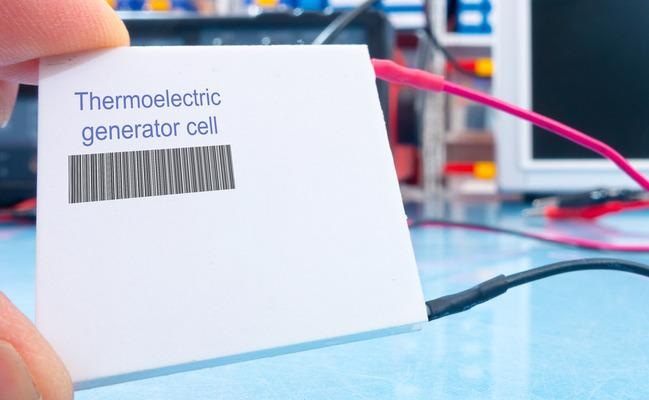
Image Credit: luchschenF/Shutterstock.com
What if this wasted heat could be captured and redirected to another function, otherwise drawing power from the grid? Addressing this question is critical. Energy needs are increasing, but fossil fuel supplies continue to dwindle. Although renewable energy is the favored alternative, it is not enough, and other means of providing energy need to be found. Only then can the effects of climate change be reduced.
Thermal energy is recoverable from operating electronic devices like computers and phones, running vehicles - even the human body. Thermoelectric energy harvesting captures thermal energy from the environment and converts it into electricity without batteries or a grid connection. It is considered the best shot for significantly reducing fossil fuels use and preventing a worldwide energy crisis.
Thermal harvesters are based on the thermoelectric effect and use thermoelectric energy generator (TEG) devices to convert heat directly into electrical energy. In this process, thermoelectric materials are utilized, which work on the principle that a temperature change across a semiconductor material creates a voltage that causes electricity to flow.
TEGs and the Seebeck Effect
TEGs generate electricity in applications where heat would otherwise be lost. They produce green energy, are relatively maintenance-free (since they have no moving parts), and are environmentally efficient, producing no greenhouse gases. Furthermore, they work on hot and cold surfaces and are scalable to capture vast amounts of energy.
These devices can be made with different technologies using several fabrication methods on materials such as silicon, which is used for small-scale micro and nanometer generators. Others include alumina, and low temperature co-fired ceramics (LTCC), which allow for a multilayered approach to high-density TEGs. Polymers including polyamide, cellulose fiber-based, and fabric are also popular.
TEGs exploit the Seebeck effect - the conversion of a temperature gradient into electrical power at the junctions of the device’s thermoelectric elements. This is limited by the thermoelectric figure of merit, ZT, of the thermoelectric material. ZT is equal to S2σT/κtot (S being the Seebeck coefficient, σ the electrical conductivity, T the absolute temperature and κtot the total thermal conductivity).
Consequently, there is a focus on improving the thermoelectric conversion efficiency of TEGs, which can be achieved by optimizing thermoelectric materials and improving ZT. The higher the ZT, the better the material and TEG. They must have a high Seebeck Coefficient to maximize energy conversion, in addition to high electrical conductivity and low thermal conductivity.
Spin and Quantum Thermoelectrics
Research from Tokyo University published in the journal Nature discovered the Spin Seebeck Effect (SSE). Here, the temperature difference between nonmagnetic and ferromagnetic material creates a flow of spins. It transpires that the inverse is important as the spin flow can be transformed into a current with an electric charge, i.e., a way to generate electricity from the inverse SSE and enhance efficiency.
Further research from Chung-Ang University in Korea published in the journal Nano Letters considered the effect of a molybdenum disulfide (MoS2) layer. The layer caused the promotion of two independent quantum phenomena that accounted for the total inverse SSE, namely the inverse spin Hall effect and the inverse Rashba-Edelstein effect - which both produce a spin accumulation that is then converted into a charge current.
Their research has improved the understanding of SSE in spin transport and could be used to investigate other types of quantum transport.
Applications and the Future
TEGs are used in the automobile industry in car engines to reduce fuel energy costs and improve engine performance. They recover heat from exhaust gases and the internal combustion engine, converting it into electrical energy. This can then be used by the navigation system, electronic breaking, telematics, and stability controls.
Waste heat is a complex issue for electronic devices like Central Processing Units (CPU) and integrated circuits; TEGs could supply other components or activate cooling fans, increasing battery performance and lifetime. They are also found in spacecraft, satellites, and probes, where heat released by natural radioactive decay is converted into electrical energy.
Closer to home, flexible, wearable TEGs are found in health monitoring and tracking devices. Specific examples include wearable textiles and garments and devices that utilize body heat to generate electricity for their power supply. For such purposes, devices must be biocompatible; they must be non-toxic to the body and are often shrouded in a special membrane before implantation.
The industry and market for TEG devices are still relatively small but are likely to proliferate in the future thanks to an increase in published research papers, more R&D in the area, and a greater demand for new thermoelectric materials and better TEG devices.
References and Further Reading
Enescu, D., (2019) Thermoelectric Energy Harvesting: Basic Principles and Applications. Green Energy Advances. Available at: https://www.intechopen.com/chapters/65239 [Accessed August 2021]
Afework, B., Hanania, J., Stenhouse, K. and Donev, J., (2018) Waste heat - Energy Education. [online] Energyeducation.ca. Available at: https://energyeducation.ca/encyclopedia/Waste_heat [Accessed August 2021]
Phys.org. (2021) From heat to spin to electricity: Understanding spin transport in thermoelectric devices. [online] Available at: https://phys.org/news/2021-01-electricity-thermoelectric-devices.html [Accessed August 2021]
Jaziri, N., Boughamoura, A., Müller, J., Mezghani, B., Tounsi, F. and Ismail, M., (2020) A comprehensive review of Thermoelectric Generators: Technologies and common applications. Energy Reports, 6, pp.264-287. Available at: https://doi.org/10.1016/j.egyr.2019.12.011 [Accessed August 2021]
Uchida, K., Takahashi, S., Harii, K., Ieda, J., Koshibae, W., Ando, K., Maekawa, S. and Saitoh, E., (2008) Observation of the spin Seebeck effect. Nature, 455(7214), pp.778-781. Available at: https://doi.org/10.1038/nature07321 [Accessed August 2021]
Lee, W., Park, N., Kim, G., Kang, M., Choi, J., Choi, K., Jang, H., Saitoh, E. and Lee, S., (2020) Enhanced Spin Seebeck Thermopower in Pt/Holey MoS2/Y3Fe5O12 Hybrid Structure. Nano Letters, 21(1), pp.189-196. Available at: https://doi.org/10.1021/acs.nanolett.0c03499 [Accessed August 2021]
Disclaimer: The views expressed here are those of the author expressed in their private capacity and do not necessarily represent the views of AZoM.com Limited T/A AZoNetwork the owner and operator of this website. This disclaimer forms part of the Terms and conditions of use of this website.