The seemingly limitless potential of the sun and solar power has inspired a huge number of technological developments to capture and convert this energy. The sun provides more energy to the surface of the Earth each day than our total worldwide energy usage. Recent reports have suggested that the ability of photovoltaics to meet our energy demands may have been greatly underestimated.1
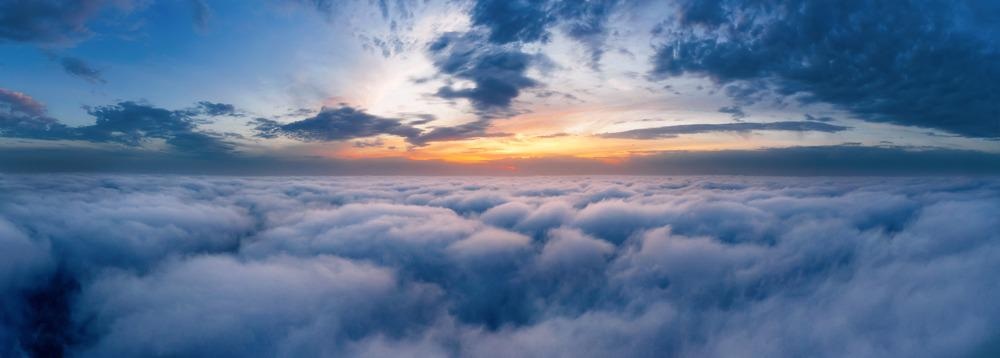
Image Credit: arturnichiporenko/Shutterstock.com
Capturing and using the sun’s energy on Earth faces several inherent challenges. The layers of our atmosphere absorb a good percentage of the incident radiation. This includes absorption of significant amounts of UV light by the ozone in the atmosphere as well as absorption of infrared wavelengths by methane, carbon dioxide and water vapor.
Some percentage of light is also lost from scattering processes due to clouds, but nearly 70% of the total light reaches the Earth’s surface. Some wavelengths of light, such as UV-C, are nearly completely blocked due to attenuation by molecular species in the atmosphere but a significant amount of visible (400 – 750 nm) and longer wavelength radiation is only somewhat attenuated.
Photovoltaics on satellites in space and objects outside of our atmosphere, therefore, have an advantage both in the total amount of light available to be absorbed and in the broader range of spectral wavelengths available. This means the theoretical limit for total energy conversion is higher. Such solar energy capture devices are also not subject to reduced input radiation because of clouding or other atmospheric changes and so can provide highly reliable energy capture and conversion.
Satellite Power
Photovoltaics have been used on spacecraft for many years, with the Vanguard I mission in 1958 being the first to carry solar cells.2 With six small cells of 10% operating efficiency, the Vanguard I was the first small step in what would become a rapid acceleration to see device efficiencies smash the 20% limit. The development of new photovoltaic architectures and technologies and solar cells become a common feature, not just on spacecraft but on Earth.
Space photovoltaics face some challenges in their design and construction to make them durable enough to survive the launch procedures and ensure they can endure the harsh environments found in space. The photovoltaic cells are often designed in combination with mirror arrays, particularly for spacecraft with high energy demands.
The mirror arrays help to capture greater amounts of light and track the position of the sun, so the photovoltaic cells can be bathed in a continual amount of light for energy conversion. In space, the lack of daily light cycles, atmospheres, and clouds means that solar energy is a truly non-stop source of power with no interruptions.
Powering the Earth with Solar Satellites
Could the greater solar energy availability in space be harnessed for use on Earth rather than just on space vehicles? Scientists have been exploring how solar satellites could potentially be used as an efficient source for the capture of radiation in space but then transmit that back to Earth via either a laser beam or through microwave radiation.
The concept would be that the space-based assemblies act as continual harvesters for light energy and then the beams transmitted from these could be sent on to dedicated receivers. This would make it possible to directly transmit energy even to remote regions without extensive electrical infrastructure and overcome the drop in electricity generation that occurs overnight from solar sources.
Researchers at the Lawrence Berkeley National Laboratories say that satellite and photovoltaic technologies have advanced sufficiently that the idea of solar energy satellites powering the Earth is now a real possibility. The proposed devices for microwave transmission of solar power are huge – more than 3 km in diameter and weighing over 80,000 metric tons.
However, such a satellite could provide gigawatts of power, enough to keep a major US city running continually. While the levels of power are appealing, the challenge with microwave-based devices is the extensive transmission area required on Earth – totaling 3-10 km.
Therefore, laser-based transmission may be a more appealing route that offers a more compact instrument requiring significantly smaller transmission areas, totaling just 2 m in area. At just 10 metric tons, a laser-based system would be significantly cheaper to design, build and launch, though multiple satellites would be required to deliver the same amount of power as the microwave monsters.
The energy transmission mechanism would affect the orbiting distance of the satellite, with laser-based devices orbiting around 400 km above Earth and microwave-based craft being located much further out - around 35,000 km. While the laser technologies for high-power alkali lasers have been demonstrated, there are still several considerations, both political and technical, before we are likely to see the presence of such satellites in our skies. However, their possibility as a constant, renewable, clean source of energy is an exciting one.
References and Further Reading
- Creutzig, F., Agoston, P., Goldschmidt, J. C., Luderer, G., Nemet, G., & Pietzcker, R. C. (2017). The underestimated potential of solar energy to mitigate climate change. Nature Energy, 2(9). https://doi.org/10.1038/nenergy.2017.140
- Bailey, S. G., & Flood, D. J. (1998). Space photovoltaics. Progress in Photovoltaics: Research and Applications, 6(1), 1–14. https://doi.org/10.1002/(sici)1099-159x(199801/02)6:1<1::aid-pip204>3.0.co;2-x
- US Department of Energy (2021) Space Energy, https://www.energy.gov/articles/space-based-solar-power
Disclaimer: The views expressed here are those of the author expressed in their private capacity and do not necessarily represent the views of AZoM.com Limited T/A AZoNetwork the owner and operator of this website. This disclaimer forms part of the Terms and conditions of use of this website.