The Antarctic Silverfish (Pleuragramma antarctica; Notothenioidei) is the only indigenous Southern Ocean fish with a fully pelagic life cycle, accounting for approximately 90% of adult and larval fish biomass in coastal parts of the Southern Ocean. Antarctic Silverfish is a significant prey for predators such as seals, penguins, seabirds, and other fishes due to their quantity and availability in the upper water column.
Climate Change Affects Antarctic Silverfish Population in Western Antarctic Peninsula" />
Image Credit: I. Noyan Yilmaz/Shutterstock.com
Antarctic silverfish have a circumpolar existence, with a genetic linkage between populations along the continental shelf. The Western Antarctic Peninsula (WAP) region (Figure 1) lacks an Antarctic Slope Front Current, and this separates the Antarctic Silverfish group from other established sites of reproduction in the species.
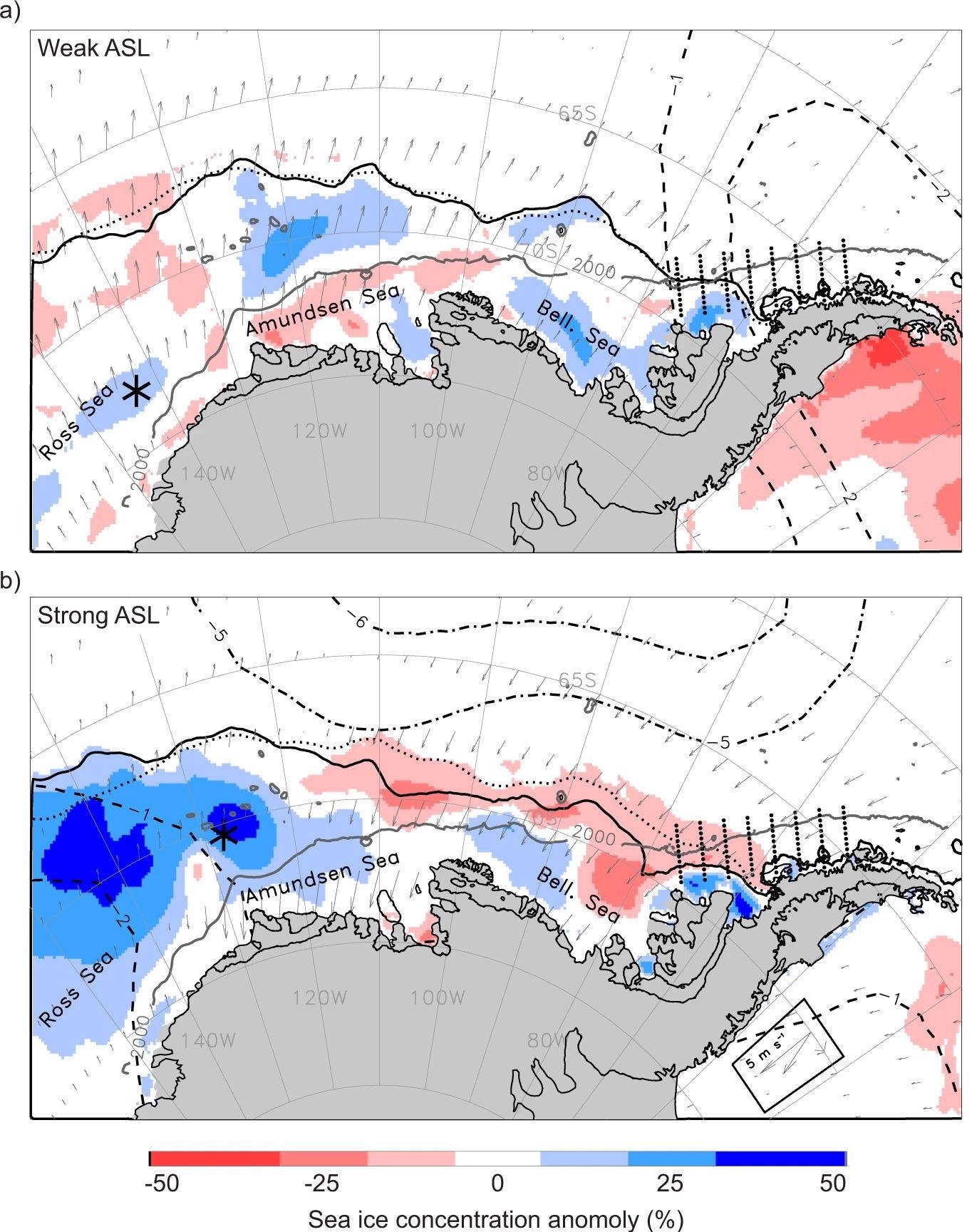
Figure 1. Impact of the Amundsen Sea Low (ASL) on the West Antarctic environment. (a) Averaged environmental conditions for the duration of an especially weak (shallow; relative central pressure, RCP, of −6) ASL event during March–April–May (MAM) 1993. The ASL central location is marked by the black asterisk and the Palmer Antarctica Long-Term Ecological Research program study region and sampling stations are depicted by eight lines of small black dots. Sea ice concentration anomalies are color shaded and the MAM 1993 mean ice edge contour (solid black line) and long-term (1979–2019) mean contour (dotted black line) are also marked. Subsampled wind anomalies are shown in vector format. The negative sea-level pressure (SLP) anomalies are shown for the most negative feature during MAM 1993 (dashed concentric contours). (b) The same averaged environmental conditions and symbology as in (a) but for the duration of an especially strong (deep; RCP of −16) ASL event during MAM 1996. The wind-vector legend for both 1993 and 1996 is boxed in the lower right corner. Image Credit: Corso, et al. 2022
The Southern Annular Mode (SAM), El Niño Southern Oscillation (ENSO), and Amundsen Sea Low (ASL) are the three atmospheric circulation patterns that have the most influence on climate change in the WAP region. Interconnections between them are complicated, seasonal, and linked to anthropogenic consequences such as global greenhouse gas emissions.
The Amundsen Sea Low (ASL), a climatologically low-pressure center in the Amundsen Sea (Figure 1), was only recently discovered as the primary driver of WAP ocean warming, sea ice loss, and glacier retreat over the past century.
The ASL’s strength and location influence meridional winds east and west of the ASL’s center (Figure 1a, b). Greater ASL events, for example, in the Amundsen Sea boost warm northerly airflow over the WAP region, reducing sea ice extent and concentration (Figure 1b) and increasing surface temperature along the WAP.
In this research, scientists looked at the interconnection between Antarctic Silverfish and the WAP environment over a two-decade period. Researchers specifically examined the effect of sea surface temperatures, sea ice dynamics, atmospheric circulation patterns, chlorophyll, and salinity on the vast quantities of larval Antarctic Silverfish. Researchers also use the findings of this study to forecast how regional pelagic food webs will respond to future climate change.
Results and Discussion
Researchers organized, recognized, and counted Antarctic Silverfish larvae (n = 7086) collected over 25 years between 1993 and 2017. Plankton net tows based on the Palmer Antarctica Long-Term Ecological Research (Palmer LTER) Program are depicted in Figure 2a.
As shown in Figure 2b, zero-inflated generalized linear mixed-effects model (GLMM) predictions show that the enormous amount of larvae during this period is closely related to ocean temperature, with higher abundance in colder water.
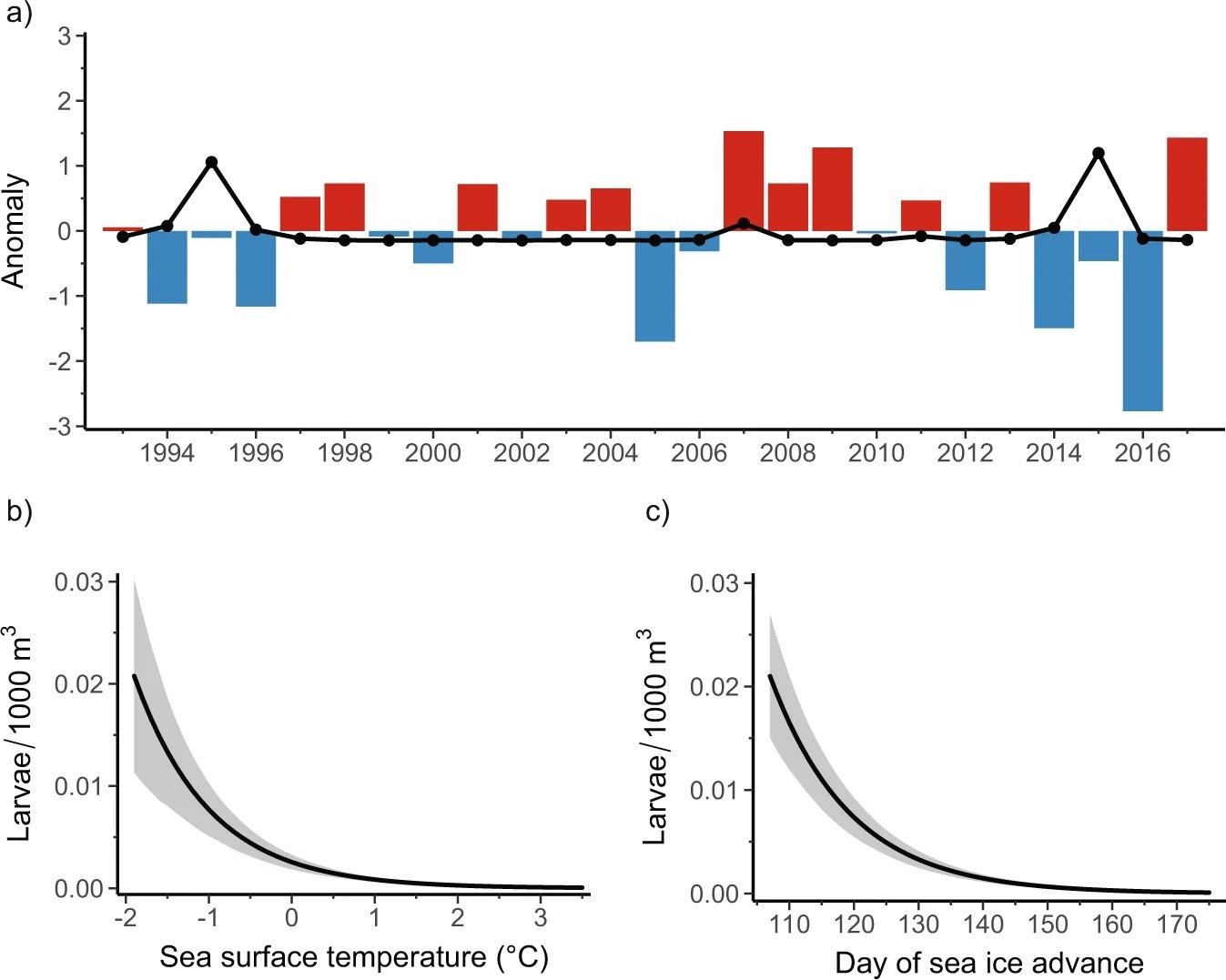
Figure 2. Relationships between sea surface temperature, sea ice, and Antarctic Silverfish abundance. (a) Positive (red; warmer temperatures) and negative (blue; cooler temperatures) anomalies in the standardized mean sea surface temperature (see “Methods”) for the Palmer LTER study region during austral summer (December, January, February). Standardized anomalies in mean annual larval Antarctic Silverfish abundance (larvae/1000 m3) that were captured during January and February are overlaid (black dotted line). (b, c) Predicted impact (solid black lines) on larval Antarctic Silverfish abundance from (b) sea surface temperature (p < 0.001) and (c) lagged day of sea ice advance (p < 0.001) from the model. Sea ice advance was temporally lagged in the model to align with life history patterns in adult Antarctic Silverfish abundance. The shaded regions represent the 95% prediction interval, which considers uncertainty from the fixed effects, zero-inflation, and random effects components of the final model. Image Credit: Corso, et al. 2022
Figure 2a shows that at least two consecutive years of abnormally cold surface temperatures are required to produce peaks in larval abundance based on the time series.
As shown in Figure 2c, the timing of sea ice advance through austral autumn (March–May) influences larval abundance the very next year.
Adult fish spawn in late austral winter to early spring (July–September), eggs grow for about four months and fry hatch in November and December (Figure 3a). Adults then improve their nutritional status for a year before reproducing the following season.
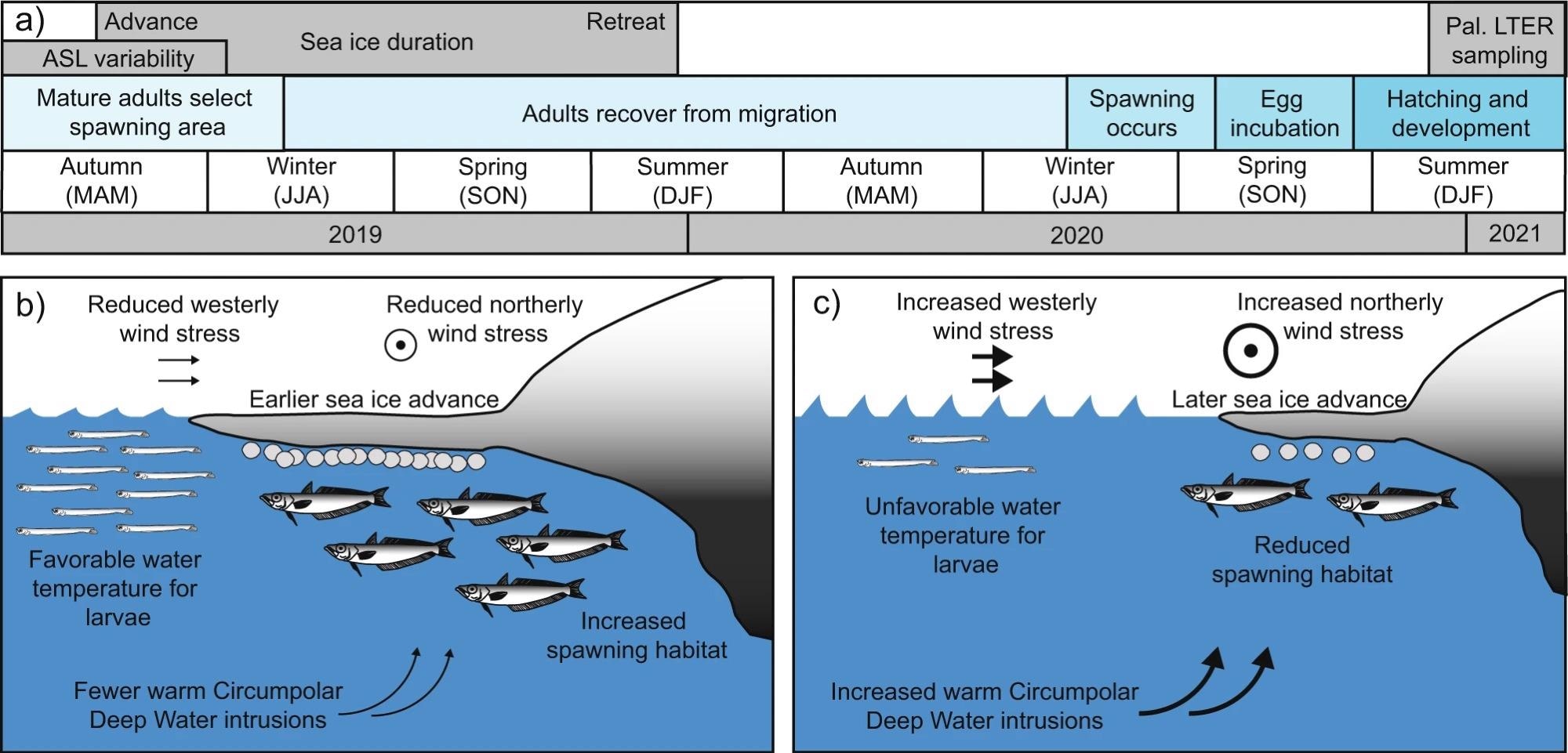
Figure 3. Timeline with optimal and suboptimal conditions for Antarctic Silverfish reproduction near the western Antarctic Peninsula (WAP). (a) A timeline of Antarctic Silverfish skip-spawning behavior and proposed relationships with Amundsen Sea Low (ASL) variability and sea ice advance. (b, c) Schematic of b optimal and c suboptimal environmental conditions for larval and spawning adult Antarctic Silverfish near the WAP. Northerly and westerly wind stresses are both modulated by the ASL strength (i.e., RCP) and location (i.e., latitude). Intrusions of Circumpolar Deep Water (CDW) are associated with anomalous westerly winds; sea ice advance is influenced by a combination of wind stress, CDW, precipitation, and other factors; and near-surface water temperatures are determined by atmospheric heat, CDW intrusions, ice melt, and other factors. Image Credit: Corso, et al. 2022
Adult Antarctic Silverfish, according to the scientists, choose their spawning area depending in part on the presence of adequate sea ice cover at the time of austral autumn (Figures 2a and 3b, c). An initial advance of sea ice in late April or early May (Figure 2c) serves as a positive signal for transferring adults and enhances spawning habitat (Figure 3b).
If the spawning season is postponed, the spawning habitat is lowered (Figure 3c), which may cause adults to travel elsewhere or persist to postpone spawning. As a result, scientists predict that sea ice advances beginning around day 157 or earlier are required for spawning to happen successfully in this region.
The enormous amount of larval Antarctic silverfish was also strongly linked with the ASL relative central pressure (RCP), which is an index of ASL strength, and the ASL’s latitudinal location (Figure 4a).
A poleward ASL location was linked to lower spawning success, as evidenced by the lower abundance of larvae recorded the very next year (Figure 4b).
![Predicted impact of Amundsen Sea Low relative central pressure and latitudinal location on larval Antarctic Silverfish abundance. (a, b) Predicted impact (solid black lines) on larval Antarctic Silverfish abundance from (a) the relative central pressure (RCP) of the Amundsen Sea Low (ASL) during austral autumn (March–April–May [MAM]; p = 0.006) and (b) the latitudinal location of the ASL during austral autumn (p = 0.004) from the model (see “Methods”). Smaller RCP values correspond with stronger (i.e., deeper) ASL events and smaller latitudinal values correspond with southward (i.e., poleward) locations of the ASL. The ASL RCPs and locations were temporally lagged in the model to align with life history patterns in adult Antarctic Silverfish abundance. The shaded regions represent the 95% prediction interval, which considers uncertainty from the fixed effects, zero-inflation, and random effects components of the final model. It is important to note only one value of ASL RCP was greater (less negative) than -4.5, which accounts for the increased uncertainty at less negative values (>-4.5). Additionally, we observed no significant collinearity between ASL RCP and latitude in this analysis.](https://d3hnfqimznafg0.cloudfront.net/images/Article_Images/ImageForArticle_1447_16454358572404390.jpg)
Figure 4. Predicted impact of Amundsen Sea Low relative central pressure and latitudinal location on larval Antarctic Silverfish abundance. (a, b) Predicted impact (solid black lines) on larval Antarctic Silverfish abundance from (a) the relative central pressure (RCP) of the Amundsen Sea Low (ASL) during austral autumn (March–April–May [MAM]; p = 0.006) and (b) the latitudinal location of the ASL during austral autumn (p = 0.004) from the model (see “Methods”). Smaller RCP values correspond with stronger (i.e., deeper) ASL events and smaller latitudinal values correspond with southward (i.e., poleward) locations of the ASL. The ASL RCPs and locations were temporally lagged in the model to align with life history patterns in adult Antarctic Silverfish abundance. The shaded regions represent the 95% prediction interval, which considers uncertainty from the fixed effects, zero-inflation, and random effects components of the final model. It is important to note only one value of ASL RCP was greater (less negative) than −4.5, which accounts for the increased uncertainty at less negative values (>−4.5). Additionally, we observed no significant collinearity between ASL RCP and latitude in this analysis. Image Credit: Corso, et al. 2022
Methodology
The larval fishes used in this research were taken in accordance with Palmer LTER program protocols and acquired as preserved specimens cataloged in the Virginia Institute of Marine Science (VIMS) Nunnally Ichthyology Collection.
Tows were conducted from the 600 grid line to the 200 grid line from 1993 to 2007. The “far south” lines (100, 000, and −100) were added to the Palmer LTER grid in 2008.
Scientists included extra tows conducted at Process Study stations located between grid lines from 1993 to 2017. The relationships remained unchanged when the researchers ran the final model without the Process Study and far south stations.
Due to the presence of two distinct dorsal pigment rows and an absence of abdominal pigmentation, Antarctic Silverfish is one of the most conveniently recognized of the known larval fishes endemic to the Southern Ocean.
Andrew D. Corso recognized the majority of the fishes to species level, and the remaining fishes were observed by Dr. Peter Konstantinidis of Oregon State University and other scientists affiliated with the VIMS Nunnally Ichthyology Collection.
Antarctic larval fishes exist in the upper 300 m of the water column. They were incidentally captured by Palmer LTER trawls targeting zooplankton (e.g., krill, salps, copepods). Depending on hypothesis testing, parsimony, and minimizing the Akaike information criterion (AIC), the final model was selected. Diagnostic residual plots were employed to analyze the model performance.
Conclusion
Since the Trophic relationships and life history of Antarctic Silverfish are mostly known from the Ross and Weddell seas, it will be crucial to further characterize this keystone fish’s role in the vulnerable WAP pelagic ecosystem.
This study also reveals the value and significance of long-term sampling programs as well as natural history collections. Curated time series of larval fishes are an important resource for determining the causes of adult population changes.
Ultimately, the ecosystem-level effects of climate change must be considered as part of air-sea interactions, such as the ASL, to more clearly foresee food web shifts and handle natural resources in the region.
Journal Reference:
Corso, A. D., Steinberg, D. K., Stammerjohn, S. E., Hilton, E. J. (2022) Climate drives long-term change in Antarctic Silverfish along the western Antarctic Peninsula. Communications Biology, 5, p. 104. Available Online: https://www.nature.com/articles/s42003-022-03042-3.
References and Further Reading
- DeWitt, H H (1970) The character of the midwater fish fauna of the Ross Sea, Antarctica. Antarctic Ecology, 1, pp. 305–314.
- Guglielmo, L, et al. (1997) Distribution and abundance of postlarval and juvenile Pleuragramma antarcticum (Pisces, Nototheniidae) off Terra Nova Bay (Ross Sea, Antarctica). Polar Biology,19, pp. 37–51. doi.org/10.1007/s003000050214.
- La Mesa, M & Eastman, J T (2012) Antarctic silverfish: life strategies of a key species in the high-Antarctic ecosystem. Fish Fisheries, 13, pp. 241–266. doi.org/10.1111/j.1467-2979.2011.00427.x.
- La Mesa, M., et al. (2004) The role of notothenioid fish in the food web of the Ross Sea shelf waters: a review. Polar Biology, 27, pp. 321–338. doi.org/10.1007/s00300-004-0599-z.
- Pinkerton, M. H., et al. (2010) A balanced model of the food web of the Ross Sea, Antarctica. CCAMLR Science, 17, pp. 1–31.
- Caccavo, J. A., et al. (2018) Along-shelf connectivity and circumpolar gene flow in Antarctic silverfish (Pleuragramma antarctica). Scientific Reports, 8, pp. 1–16. doi.org/10.1038/s41598-018-36030-x.
- Beers, J M &Jayasundara, N (2015) Antarctic notothenioid fish: what are the future consequences of ‘losses’ and ‘gains’ acquired during long-term evolution at cold and stable temperatures? Journal of Experimental Biology, 218, pp. 1834–1845. doi.org/10.1242/jeb.116129.
- Bilyk, K T &DeVries, A L (2011) Heat tolerance and its plasticity in Antarctic fishes. Comparative Biochemistry & Physiology, A Molecular and Integrative Physiology, 158, pp. 382–390. doi.org/10.1016/j.cbpa.2010.12.010.
- Sandersfeld, T., et al. (2015) Elevated temperature causes metabolic trade-offs at the whole-organism level in the Antarctic fish Trematomusbernacchii. Journal of Experimental Biology, 218, pp. 2373–2381. doi.org/10.1242/jeb.122804.
- Cook, A. J., et al. (2016) Ocean forcing of glacier retreat in the western Antarctic Peninsula. Science, 353, pp. 283–286. doi.org/10.1126/science.aae0017.
- Stammerjohn, S E & Scambos, T A (2020) Warming reaches the South Pole. Nature Climate Change,10, pp. 710–711. doi.org/10.1038/s41558-020-0827-8.
- Henley, S. F., et al. (2019) Variability and change in the west Antarctic Peninsula marine system: research priorities and opportunities. Progress in Oceanography, 173, pp. 208–237. doi.org/10.1016/j.pocean.2019.03.003.
- Mintenbeck, K & Torres, J J (2017) in The Antarctic silverfish: a keystone species in a changing ecosystem, pp. 253–286 (Springer).
- Vacchi, M., et al. (2012) A nursery area for the Antarctic silverfish Pleuragrammaantarcticum at Terra Nova Bay (Ross Sea): first estimate of distribution and abundance of eggs and larvae under the seasonal sea-ice. Polar Biology, 35, pp. 1573–1585. doi.org/10.1007/s00300-012-1199-y.
- Vacchi, M., et al. (2004) Early life stages in the life cycle of Antarctic silverfish, Pleuragrammaantarcticum in Terra Nova Bay, Ross Sea. Antarctic Science, 16, pp. 299–305. doi.org/10.1017/S0954102004002135.
- Kellermann, A K (1996) Midwater fish ecology. FoundEcological Research. West Antarctic Peninsula, 70, pp. 231–256. https://agupubs.onlinelibrary.wiley.com/doi/abs/10.1029/AR070p0231.
- La Mesa, M., et al. (2015) Reproductive resilience of ice-dependent Antarctic silverfish in a rapidly changing system along the Western Antarctic Peninsula. Marine Ecology,36, pp. 235–245. doi.org/10.1111/maec.12140.
- Parker, M. L., et al. (2015) Assemblages of micronektonic fishes and invertebrates in a gradient of regional warming along the Western Antarctic Peninsula. Journal of Marine Systems,152, pp. 18–41.
- Ross, R. M., et al. (2014) Trends, cycles, interannual variability for three pelagic species west of the Antarctic Peninsula 1993–2008. Marine Ecology Progress Series, 515, pp. 11–32. doi.org/10.3354/meps10965.
- Koubbi, P. et al. (2011) Spatial distribution and inter-annual variations in the size frequency distribution and abundances of Pleuragramma antarcticum larvae in the Dumont d’Urville Sea from 2004 to 2010. Polar Biology,5, pp. 225–238.
- Davis, L. B., et al. (2017) Distributions of krill and Antarctic silverfish and correlations with environmental variables in the western Ross Sea, Antarctica. Marine Ecology Progress Series, 584, pp. 45–65. doi.org/10.3354/meps12347.
- La Mesa, M., et al. (2010) Influence of environmental conditions on spatial distribution and abundance of early life stages of Antarctic silverfish, Pleuragramma antarcticum (Nototheniidae), in the Ross Sea. Antarctic Science,22, p. 243. doi.org/10.1017/S0954102009990721.
- Raphael, M. N., et al. (2016) The Amundsen Sea low: variability, change, and impact on Antarctic climate. Bulletin of the American Meteorological Society, 97, pp. 111–121. doi.org/10.1175/BAMS-D-14-00018.1.
- Fogt, R. L., et al. (2012) The characteristic variability and connection to the underlying synoptic activity of the Amundsen-Bellingshausen Seas Low. Journal of Geophysical Research Atmospheres. doi.org/10.1029/2011JD017337.
- Hosking, J. S., et al. (2013) The influence of the Amundsen–Bellingshausen Seas low on the climate of West Antarctica and its representation in coupled climate model simulations. Journal of Climate,26, pp. 6633–6648. doi.org/10.1175/JCLI-D-12-00813.1.
- Hosking, J. S., et al. (2016) Future circulation changes off West Antarctica: sensitivity of the Amundsen Sea Low to projected anthropogenic forcing. Geophysical Research Letters,43, pp. 367–376.
- Hobbs, W. R., et al. (2016) A review of recent changes in Southern Ocean sea ice, their drivers and forcings. Global and Planetary Change,143, pp. 228–250.
- Stammerjohn, S. E., et al. (2015) Seasonal sea ice changes in the Amundsen Sea, Antarctica, over the period of 1979–2014. Elementa: Science of the Anthropocene,3, p. 000055. doi.org/10.12952/journal.elementa.000055.
- Holland, M. M., et al. (2018) The regional, seasonal, and lagged influence of the Amundsen Sea Low on Antarctic sea ice. Geophysical Research Letters,45, pp. 11–227. doi.org/10.1029/2018GL080140.
- Thoma, M., et al. (2008) Modelling circumpolar deep water intrusions on the Amundsen Sea continental shelf, Antarctica. Geophysical Research Letters. doi.org/10.1029/2008GL034939.
- Dotto, T. S., et al. (2020) Control of the oceanic heat content of the Getz‐Dotson Trough, Antarctica, by the Amundsen Sea Low. Journal of Geophysical Research: Oceans, 125, p. e2020JC016113. doi.org/10.1029/2020JC016113.
- Holland, P. R., et al. (2019) West Antarctic ice loss influenced by internal climate variability and anthropogenic forcing. Nature Geoscience,12, pp. 718–724. doi.org/10.1038/s41561-019-0420-9.
- Dinniman, M. S., et al. (2012) Sensitivity of circumpolar deep water transport and Ice Shelf Basal Melt along the West Antarctic Peninsula to changes in the winds. Journal of Climate, 25, pp. 4799–4816. doi.org/10.1175/JCLI-D-11-00307.1.
- Dinniman, M. S., et al. (2011) A model study of circumpolar deep water on the West Antarctic Peninsula and Ross Sea continental shelves. Deep-Sea Research Part II: Topical Studies in Oceanography,58, pp. 1508–1523. doi.org/10.1016/j.dsr2.2010.11.013.
- Nakayama, Y., et al. (2018) Origin of circumpolar deep water intruding onto the Amundsen and Bellingshausen Sea continental shelves. Nature Communications, 9, p. 3403. doi.org/10.1038/s41467-018-05813-1.
- Spence, P., et al. (2014) Rapid subsurface warming and circulation changes of Antarctic coastal waters by poleward shifting winds. Geophysical Research Letters, 41, pp. 4601–4610. doi.org/10.1002/2014GL060613.
- Greaves, B. L., et al. (2020) The Southern Annular Mode (SAM) influences phytoplankton communities in the seasonal ice zone of the Southern Ocean. Biogeosciences, 17, pp. 3815–3835. doi.org/10.5194/bg-17-3815-2020.
- Steinberg, D. K., et al. (2015) Long-term (1993–2013) changes in macrozooplankton off the Western Antarctic Peninsula. Deep Sea Research Part A. Oceanographic Research Papers,101, pp. 54–70. doi.org/10.1016/j.dsr.2015.02.009.
- La, H. S., et al. (2019) Zooplankton and micronekton respond to climate fluctuations in the Amundsen Sea polynya, Antarctica. Scientific Reports, 9, p. 10087. doi.org/10.1038/s41598-019-46423-1.
- Granata, A., et al. (2009) Summer and spring trophic niche of larval and juvenile Pleuragrammaantarcticum in the Western Ross Sea, Antarctica. Polar Biology, 32, pp. 369–382. doi.org/10.1007/s00300-008-0551-8.
- Bhaskaran, K., et al. (2013) Time series regression studies in environmental epidemiology. International Journal of Epidemiology,42, pp. 1187–1195. doi.org/10.1093/ije/dyt092.
- Ghigliotti, L., et al. (2017) Reproductive features of the Antarctic silverfish (Pleuragrammaantarctica) from the western Ross Sea. Polar Biology, 40, pp. 199–211. doi.org/10.1007/s00300-016-1945-7.
- Chapman, E. W., et al. (2011) Marine and terrestrial factors affecting Adélie penguin Pygoscelisadeliae chick growth and recruitment off the western Antarctic Peninsula. Marine Ecology Progress Series,436, pp. 273–289. doi.org/10.3354/meps09242.
- Coggins, J H J & McDonald, A J (2015) The influence of the Amundsen Sea Low on the winds in the Ross Sea and surroundings: Insights from a synoptic climatology. Journal of Geophysical Research: Atmospheres, 120, 2167–2189.
- Assmann, K. M. et al. (2013) Variability of circumpolar deep water transport onto the Amundsen Sea Continental shelf through a shelf break trough. Journal of Geophysical Research Oceans, 118, pp. 6603–6620. doi.org/10.1002/2013JC008871.
- Moffat, C., et al. (2009) On the characteristics of circumpolar deep water intrusions to the west Antarctic Peninsula Continental Shelf. Journal of Geophysical Research Oceans. doi.org/10.1029/2008JC004955.
- Dahlke, F. T., et al. (2020) Thermal bottlenecks in the life cycle define climate vulnerability of fish. Science,369, pp. 65–70. doi.org/10.1126/science.aaz3658.
- Regan, H. C., et al. (2018) Sources, variability and fate of freshwater in the Bellingshausen Sea, Antarctica. Deep-Sea Research Part I: Oceanographic Research Papers, 133, pp. 59–71. doi.org/10.1016/j.dsr.2018.01.005.
- Holland, P. R., et al. (2014) Modeled trends in Antarctic sea ice thickness. Journal of Climate, 27, pp. 3784–3801. doi.org/10.1175/JCLI-D-13-00301.1.
- Hoppmann, M., et al. (2020) Platelet ice, the Southern Ocean’s hidden ice: a review. Annals of Glaciology, 61, pp. 341–368. doi.org/10.1017/aog.2020.54.
- Arrigo, K R (2014) Sea ice ecosystems. Annual Review of Marine Science,6, pp. 439–467. doi.org/10.1146/annurev-marine-010213-135103.
- Veazey, A. L., et al. (1994) Small-scale variability of physical properties and structural characteristics of Antarctic fast ice. Annals of Glaciology, 20, pp. 61–66. doi.org/10.3189/1994AoG20-1-61-66.
- Garrison, D. L., et al. (1983) A physical mechanism for establishing algal populations in frazil ice. Nature, 306, pp. 363–365. doi.org/10.1038/306363a0.
- Quetin, L B & Ross, R M (2009) in Smithsonian at the Poles: Contributions to International Polar Year Science (edsKrupnik, I., Lang, M. A. & Miller, S. E.) pp. 285–298 (IPY). doi.org/10.5479/si.097884601X.0.
- Meredith, M P & King, J C (2005)Rapid climate change in the ocean west of the Antarctic Peninsula during the second half of the 20th century. Geophysical Research Letters, doi.org/10.1029/2005GL024042.
- Turner, J.,et al. (2016)Absence of 21st century warming on Antarctic Peninsula consistent with natural variability. Nature,535, pp. 411–415. doi.org/10.1038/nature18645.
- Rintoul, S. R.,et al. (2018)Choosing the future of Antarctica. Nature,558, pp. 233–241. doi.org/10.1038/s41586-018-0173-4.
- Turner, J.,et al. (2013)The Amundsen Sea low. International Journal of Climatology,33, pp. 1818–1829. doi.org/10.1002/joc.3558.
- Ding, Q., et al. (2011) Winter warming in West Antarctica caused by central tropical Pacific warming. Nature Geoscience,4, pp. 398–403. doi.org/10.1038/ngeo1129.
- Moline, M. A., et al. (2004) Alteration of the food web along the Antarctic Peninsula in response to a regional warming trend. Global Change Biology, 10, pp. 1973–1980. doi.org/10.1111/j.1365-2486.2004.00825.x.
- Gleiber, M (2014) Long-Term Change in Copepod Community Structure in the Western Antarctic Peninsula: Linkage to Climate and Implications for Carbon Cycling. Dissertations, Theses, and Masters Projects, College of William and Mary, Virginia Institute of Marine Science.
- Wöhrmann, A. P., et al. (1997) Adaptations of the Antarctic silverfish Pleuragramma antarcticum (Pisces: Nototheniidae) to pelagic life in high-Antarctic waters. Marine Ecology Progress Series, 151, pp. 205–218. doi.org/10.3354/meps151205.
- Venables, H. J., et al. (2013) Wintertime controls on summer stratification and productivity at the western Antarctic Peninsula. Limnology and Oceanography,58, pp. 1035–1047. doi.org/10.4319/lo.2013.58.3.1035.
- Meredith, M. P., et al. (2008) Variability in the freshwater balance of northern Marguerite Bay, Antarctic Peninsula: results from δ18O. Deep-Sea Research Part II: Topical Studies in Oceanography,55, pp. 309–322. doi.org/10.1016/j.dsr2.2007.11.005
- Slosarczyk, W (1986) Attempts at a quantitative estimate by trawl sampling of distribution of postlarval and juvenile notothenioids (Pisces, Perciformes) in relation to environmental conditions in the Antarctic Peninsula region during SIBEX 1983–84. Memoirs of National Institute of Polar Research. Special issue, 40, pp. 299–315.
- Varsamos, S., et al. (2005) Ontogeny of osmoregulation in postembryonic fish: a review. Comparative Biochemistry & Physiology,Part A: Molecular & Integrative Physiology, 141, pp. 401–429. doi.org/10.1016/j.cbpb.2005.01.013.
- Gille, S. T., et al. (2016) Temporal changes in the Antarctic circumpolar current: implications for the Antarctic Continental Shelves. Oceanography, 29, pp. 96–105. doi.org/10.5670/oceanog.2016.102.
- Thompson, D. W. J., et al. (2011) Signatures of the Antarctic ozone hole in Southern Hemisphere surface climate change. Nature Geoscience,4, pp. 741–749. doi.org/10.1038/ngeo1296.
- Allen, M., et al. (2019) Technical summary: global warming of 1.5 °C. An IPCC Special Report on the impacts of global warming of 1.5 °C above pre-industrial levels and related global greenhouse gas emission pathways, in the context of strengthening the global response to the threat of climate change, sustainable development, and efforts to eradicate poverty. Available at: https://www.ipcc.ch/site/assets/uploads/sites/2/2018/12/SR15_TS_High_Res.pdf.
- Screen, J. A., et al. (2018) Polar climate change as manifest in atmospheric circulation. Current Climate Change Reports, 4, pp. 383–395. doi.org/10.1007/s40641-018-0111-4.
- Gao, M., et al. (2021) Historical fidelity and future change of Amundsen Sea Low under 1.5 °C–4 °C global warming in CMIP6. Atmospheric Research, 255, p. 105533. doi.org/10.1016/j.atmosres.2021.105533.
- Emslie, S D & McDaniel, J D (2002) Adélie penguin diet and climate change during the middle to late Holocene in northern Marguerite Bay, Antarctic Peninsula. Polar Biology, 25, pp. 222–229. doi.org/10.1007/s00300-001-0334-y.
- Fraser, W R &Trivelpiece, W Z (1996) Factors controlling the distribution of seabirds: winter-summer heterogeneity in the distribution of Adélie penguin populations. In Foundations for Ecological Research West of the Antarctic Peninsula, pp. 257–272 (American Geophysical Union).
- Cimino, M. A., et al. (2016) Projected asymmetric response of Adélie penguins to Antarctic climate change. Scientific Reports, 6, p. 28785. doi.org/10.1038/srep28785.
- Ainley, D. G., et al. (2018) Post-fledging survival of Adélie penguins at multiple colonies: chicks raised on fish do well. Marine Ecology Progress Series,601, pp. 239–251.
- Ruck, K. E., et al. (2014) Regional differences in quality of krill and fish as prey along the Western Antarctic Peninsula. Marine Ecology Progress Series,509, pp. 39–55. doi.org/10.3354/meps10868.
- Ainley, D. G., et al. (2013) Decadal trends in abundance, size and condition of Antarctic toothfish in McMurdo Sound, Antarctica, 1972–2011. Fish Fisheries,14, pp. 343–363. doi.org/10.1111/j.1467-2979.2012.00474.x.
- Eastman, J T (1985) Pleuragramma antarcticum (Pisces, Nototheniidae) as food for other fishes in McMurdo Sound, Antarctica. Polar Biology,4, pp. 155–160. doi.org/10.1007/BF00263878.
- Hanchet, S., et al. (2015) The Antarctic toothfish (Dissostichusmawsoni): biology, ecology, and life history in the Ross Sea region. Hydrobiologia,761, pp. 397–414. doi.org/10.1007/s10750-015-2435-6.
- Pinkerton, M., et al. (2007) Finding the role of Antarctic toothfish in the Ross Sea ecosystem. Water Atmos, 15, pp. 20–21.
- Hanchet, S M & Rickard, G J (2008) A hypothetical life cycle for Antarctic toothfish (Dissostichusmawsoni) in the Ross Sea region. CCAMLR Science, 15, pp. 35–53.
- Fuiman, L., et al. (2002) Behavior of midwater fishes under the Antarctic ice: observations by a predator. Marine Biology, 140, pp. 815–822. doi.org/10.1007/s00227-001-0752-y.
- Casaux, R., et al. (2006) The diet of the Weddell Seal Leptonychotes weddellii at the Danco Coast, Antarctic Peninsula. Polar Biology, 29, pp. 257–262. doi.org/10.1007/s00300-005-0048-7.
- Ponganis, P J &Stockard, T K (2007) Short note: the Antarctic toothfish: how common a prey for Weddell seals? Antarctic Science, 19, pp. 441–442. doi.org/10.1017/S0954102007000715.
- Rumolo, P., et al. (2020) The diet of Weddell seals (Leptonychotesweddellii) in Terra Nova Bay using stable isotope analysis. European Zoological Journal,87, pp. 94–104. doi.org/10.1080/24750263.2020.1720832.
- Hubold, G &Ekau, W (1990) Feeding patterns of post-larval and juvenile notothenioids in the southern Weddell Sea (Antarctica). Polar Biology, 10, pp. 255–260. doi.org/10.1007/BF00238422.
- Moreno, C., et al. (1986) The trophic niche of Pleuragramma antarcticum in the Bransfield Strait, Antarctica: quantitative comparison with other areas of the Southern Ocean. ServientCientINACH, 35, pp. 101–117.
- Gleiber, M. R., et al. (2016) Copepod summer grazing and fecal pellet production along the Western Antarctic Peninsula. Journal of Plankton Research, 38, pp. 732–750. doi.org/10.1093/plankt/fbv070.
- Garzio, L., et al. (2013) Microzooplankton grazing along the Western Antarctic Peninsula. Aquatic Microbial Ecology, 70, pp. 215–232. doi.org/10.3354/ame01655.
- Hobbie, J E (2003) Scientific accomplishments of the Long Term Ecological Research Program: an introduction. Bioscience,53, pp. 17–20. doi.org/10.1641/0006-3568(2003)053[0017:SAOTLT]2.0.CO;2.
- Hughes, B. B., et al. (2017) Long-term studies contribute disproportionately to ecology and policy. Bioscience,67, pp. 271–281. doi.org/10.1093/biosci/biw185.
- Hilton, E. J., et al. (2021) The expanding role of natural history collections. Ichthyology & Herpetology,109, pp. 379–391. doi.org/10.1643/t2020018.
- Hoey, J. A., et al. (2020) Using multiple natural tags provides evidence for extensive larval dispersal across space and through time in summer flounder. Molecular Ecology, 29, pp. 1421–1435. doi.org/10.1111/mec.15414.
- Houde, E D (2008) Emerging from Hjort’s shadow. Journal of Northwest Atlantic Fishery Science, 41, pp. 53–70. doi.org/10.2960/J.v41.m634.
- Ducklow, H. W., et al. (2007) Marine pelagic ecosystems: the West Antarctic Peninsula. Philosophical Transactions of the Royal Society B: Biological Sciences,362, pp. 67–94. doi.org/10.1098/rstb.2006.1955.
- Smith, R. C., et al. (1995) The Palmer LTER: a long-term ecological research program at Palmer Station, Antarctica. Oceanography, 8, pp. 77–86.
- Kellermann, A K (1990) Identification key and catalogue of larval Antarctic fishes. Ber. Polarforsch, pp. 1–138.
- Stammerjohn, S. E., et al. (2008) Sea ice in the western Antarctic Peninsula region: spatio-temporal variability from ecological and climate change perspectives. Deep-Sea Research Part II: Topical Studies in Oceanography,55, pp. 2041–2058. https://doi.org/10.1016/j.dsr2.2008.04.026.
- Hurrell, J W (1995) Decadal trends in the North Atlantic oscillation: regional temperatures and precipitation. Science, 269, pp. 676–679. doi.org/10.1126/science.269.5224.676.
- Hosking, J S (2020) & National Center for Atmospheric Research Staff. (eds) The Climate Data Guide: Amundsen Sea Low indices. Available at: https://climatedataguide.ucar.edu/climate-data/amundsen-sea-low-indices.
- O’Brien, R M (2007) A caution regarding rules of thumb for variance inflation factors. Qual quant, 41, pp. 673–690. doi.org/10.1007/s11135-006-9018-6.
- Gareth, J., et al. (2013) An Introduction to Statistical Learning: With Applications in R (Spinger).
- Shono, H (2008) Application of the Tweedie distribution to zero-catch data in CPUE analysis. Fisheries Research,93, pp. 154–162. doi.org/10.1016/j.fishres.2008.03.006.
- R Core Team. R (2020) A Language and Environment for Statistical Computing (R Foundation for Statistical Computing).
- Denes, F. V., et al. (2015) Estimating abundance of unmarked animal populations: accounting for imperfect detection and other sources of zero inflation. Methods in Ecology and Evolution,6, pp. 543–556. doi.org/10.1111/2041-210X.12333.
- Zuur, A F & Ieno, E N (2016) Beginner´s Guide to Zero-inflated Models with R (Highland Statistics Ltd.)
- Barnett, A. G., et al. (2010) Using information criteria to select the correct variance–covariance structure for longitudinal data in ecology. Methods in Ecology and Evolution,1, pp. 15–24. doi.org/10.1111/j.2041-210X.2009.00009.x.
- Clark, I (2010) Statistics or geostatistics? Sampling error or nugget effect? Journal of the Southern African Institute of Mining and Metallurgy, 110, pp. 307–312.
- Gschlößl, S &Czado, C (2008) Modelling count data with overdispersion and spatial effects. Statistical Papers, 49, pp. 531–552. doi.org/10.1007/s00362-006-0031-6.
- Brooks, M. E., et al. (2017) glmmTMB balances speed and flexibility among packages for zero-inflated generalized linear mixed modelling. R journal, 9, pp. 378–400. doi.org/10.3929/ethz-b-000240890.
- Aho, K., et al. (2014) Model selection for ecologists: the worldviews of AIC and BIC. Ecology,95, pp. 631–636. doi.org/10.1890/13-1452.1.
- Lüdecke, D (2018) ggeffects: Tidy data frames of marginal effects from regression models. JOSS, 3, p. 772. doi.org/10.21105/joss.00772.
- Francq, B. G., et al. (2019) Confidence, prediction, and tolerance in linear mixed models. Statistics in Medicine,38, pp. 5603–5622. doi.org/10.1002/sim.8386.
- Spineli, L M &Pandis, N (2020) Prediction interval in random-effects meta-analysis. American Journal of Orthodontics and Dentofacial Orthopedics, 157, pp.586–588. doi.org/10.1016/j.ajodo.2019.12.011.
- Comiso, J C (2000) Variability and trends in Antarctic surface temperatures from in situ and satellite infrared measurements. Journal of Climate, 13, pp. 1674–1696. doi.org/10.1175/1520-0442(2000)013%3C1674:VATIAS%3E2.0.CO;2.
- Comiso, J C &Nishio, F (2008) Trends in the sea ice cover using enhanced and compatible AMSR-E, SSM/I, and SMMR data. Journal of Geophysical Research: Oceans. doi.org/10.1029/2007JC004257.
- Hersbach, H., et al. (2019) ERA5 monthly averaged data on single levels from 1979 to present. doi.org/10.24381/CDS.F17050D7.
- Reynolds, R. W., et al. (2002) An improved in situ and satellite SST analysis for climate. Journal of Climate, 15, pp. 1609–1625. doi.org/10.1175/1520-0442(2002)015%3C1609:AIISAS%3E2.0.CO;2.