Hydrogen—a clean energy source—potentially replaces fossil fuels in a hydrogen economy, therefore, alleviating climate change. One of the main hindrances in the transition to a decarbonized energy society is the lack of storage space for industrial-scale hydrogen (H2). This article looks at Keshavarz et al.'s study looking at hydrogen diffusion in coal and its implications for hydrogen geo‐storage. The research was published in Journal of Colloid and Interface Science.
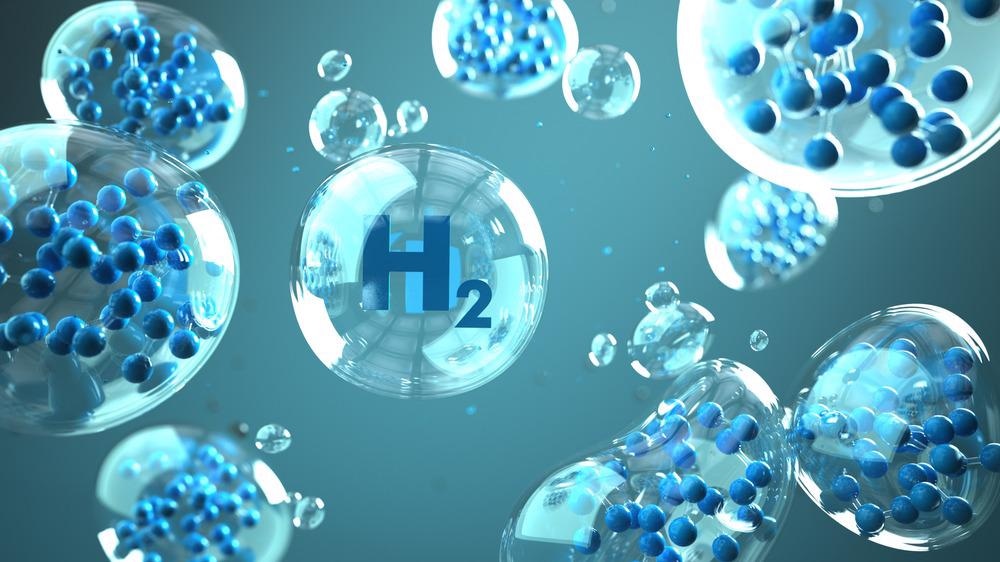
Image Credit: Alexander Limbach/Shutterstock.com
It is important to find new solutions for widespread hydrogen storage. Hydrogen underground storage (UHS) is one option that has been suggested in this context. Here, H2 is stored in underground geological formations, such as salt caverns or depleted oil and gas reservoirs.
In the recent research, the team studied the behavior of hydrogen adsorption in an Australian sub-bituminous coal sample. In the current literature, however, no data is available for hydrogen adsorption rate or diffusion kinetics. Hence, in this work, the researchers quantified such kinetic parameters in an Australian coal sample. This study offers basic petrophysical data for UHS and thereby helps in the large-scale hydrogen economy implementation.
Methodology
On an Australian anthracite sample, H2 and CO2 kinetic sorption tests were conducted. Using a blade grinder, the coal sample was ground to <500 µm. A size fraction of 250–500 µm was employed for the kinetic experiments. The coal was completely analyzed, and final and proximate analyses, helium density, and petrographic analyses findings are presented in Table 1.
Table 1. Essential analysis properties of the tested Australian anthracite coal sample. Source: Keshavarz, et al., 2022
Approximate Analysis |
Moisture Content (wt%) |
Ash Content
(wt%) |
Volatile Content
(wt%) |
Fixed Carbon
(wt%) |
Carbonate Carbon |
2.5 |
13.8 |
13.8 |
69.9 |
0.048 |
Petrographic Analysis |
Vitrinite
(Vol %) |
Liptinite
(Vol %) |
Inertinite
(Vol %) |
Mineral Matter
(Vol %) |
Vitrinite Reflectance
(Rv, max %) |
58.7 |
– |
33.3 |
8 |
4.86 |
Ultimate Analysis |
Carbon (wt%) |
Hydrogen (wt%) |
Nitrogen (wt%) sulfur |
Sulfur |
Oxygen (wt%) |
Relative
Density |
77.7 |
2.58 |
0.94 |
0.54 |
18.24 |
1.54 |
The experimental setup’s schematic view is illustrated in Figure 1.
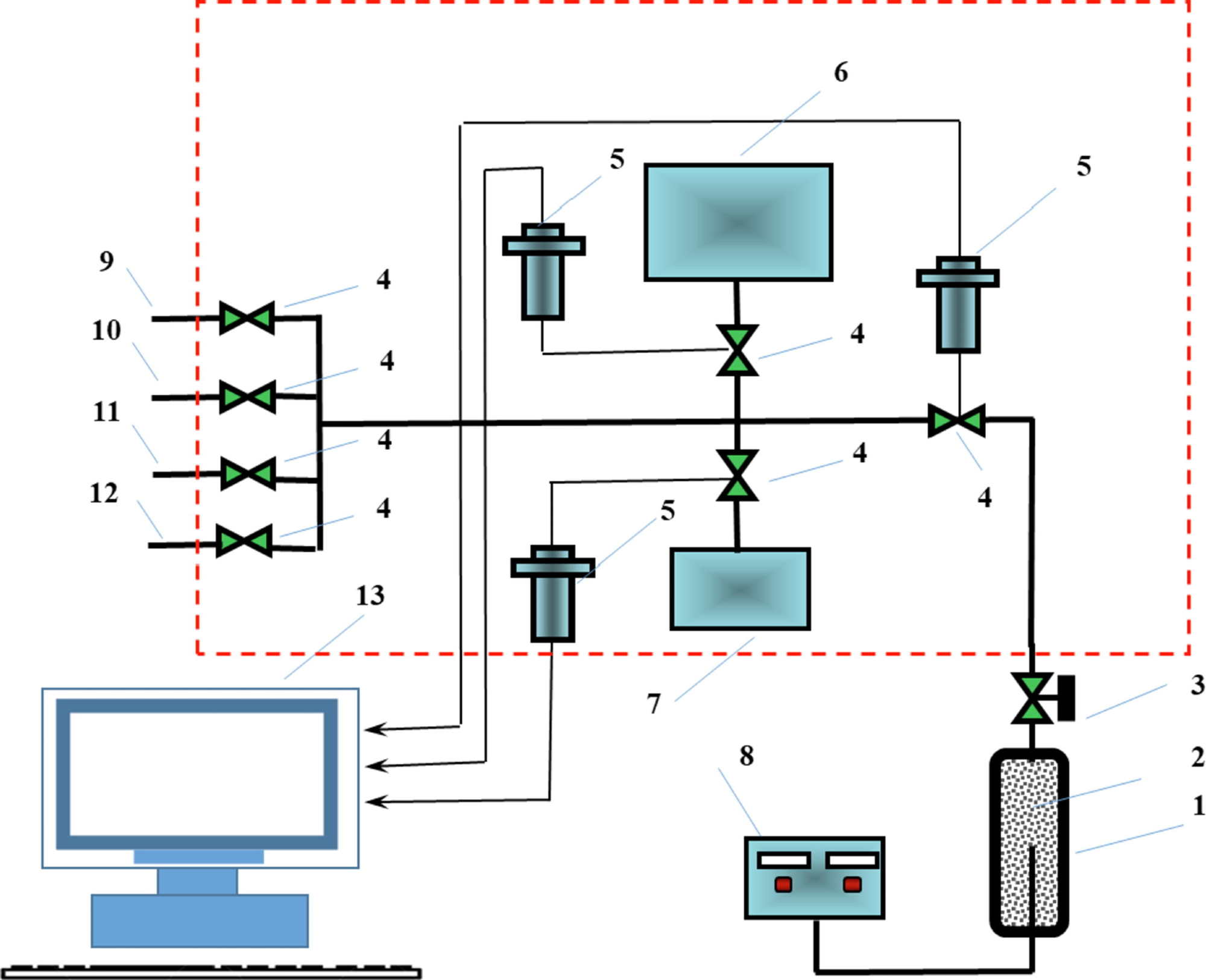
Figure. 1. Experimental set up: 1. Sample cell; 2. Coal sample; 3. Manual valve; 4. Automatic valves; 5. Pressure transducers; 6. Big reference cell; 7. Small reference cell; 8. Temperature controller; 9. Vacuum line; 10. Vent line; 11. Test gas line; 12. Calibration gas line; 13. Control panel and data acquisition system. Image Credit: Keshavarz, et al., 2017
In Table 2, parameters linked to H2, and CO2 diffusion are tabulated.
Table 2. H2 and CO2 diffusion parameters and sorption capacities, at equilibrium pressure (approximately 13 bar), for the tested coal at different temperatures. Source: Keshavarz, et al., 2022
T (°C) |
H2 |
β |
1/t0 (s−1) |
D (m2/s) 10-9 |
RMS* |
sorption capacity at equilibrium pressure (12.78–13.03 bar) |
20 |
0.34 |
0.0702 |
0.9866 |
0.02 |
0.23 |
20 |
0.33 |
0.0936 |
1.3159 |
0.02 |
0.26 |
20 |
0.35 |
0.1113 |
1.5657 |
0.02 |
0.22 |
30 |
0.33 |
0.1145 |
1.6096 |
0.02 |
0.28 |
30 |
0.35 |
0.1223 |
1.7195 |
0.01 |
0.28 |
30 |
0.39 |
0.1865 |
2.6224 |
0.01 |
0.24 |
45 |
0.40 |
0.2756 |
3.8756 |
0.01 |
0.23 |
60 |
0.39 |
0.4819 |
6.7762 |
0.01 |
0.21 |
|
CO2 |
20 |
0.32 |
0.0114 |
0.1607 |
0.02 |
1.22 |
30 |
0.37 |
0.0132 |
0.1852 |
0.02 |
1.21 |
45 |
0.35 |
0.0163 |
0.2297 |
0.01 |
1.28 |
45 |
0.40 |
0.0215 |
0.3027 |
0.02 |
1.18 |
45 |
0.40 |
0.0207 |
0.2904 |
0.01 |
1.00 |
60 |
0.35 |
0.0226 |
0.3174 |
0.02 |
1.10 |
60 |
0.36 |
0.0246 |
0.3465 |
0.02 |
1.09 |
60 |
0.37 |
0.0188 |
0.2648 |
0.02 |
1.09 |
Results and Discussion
Evidently, adsorption of H2 and CO2 reached equilibrium quicker at a higher temperature, as anticipated (Figure 2). Such quicker equilibration was made to occur by the improved gas molecular kinetic energy at greater temperatures.
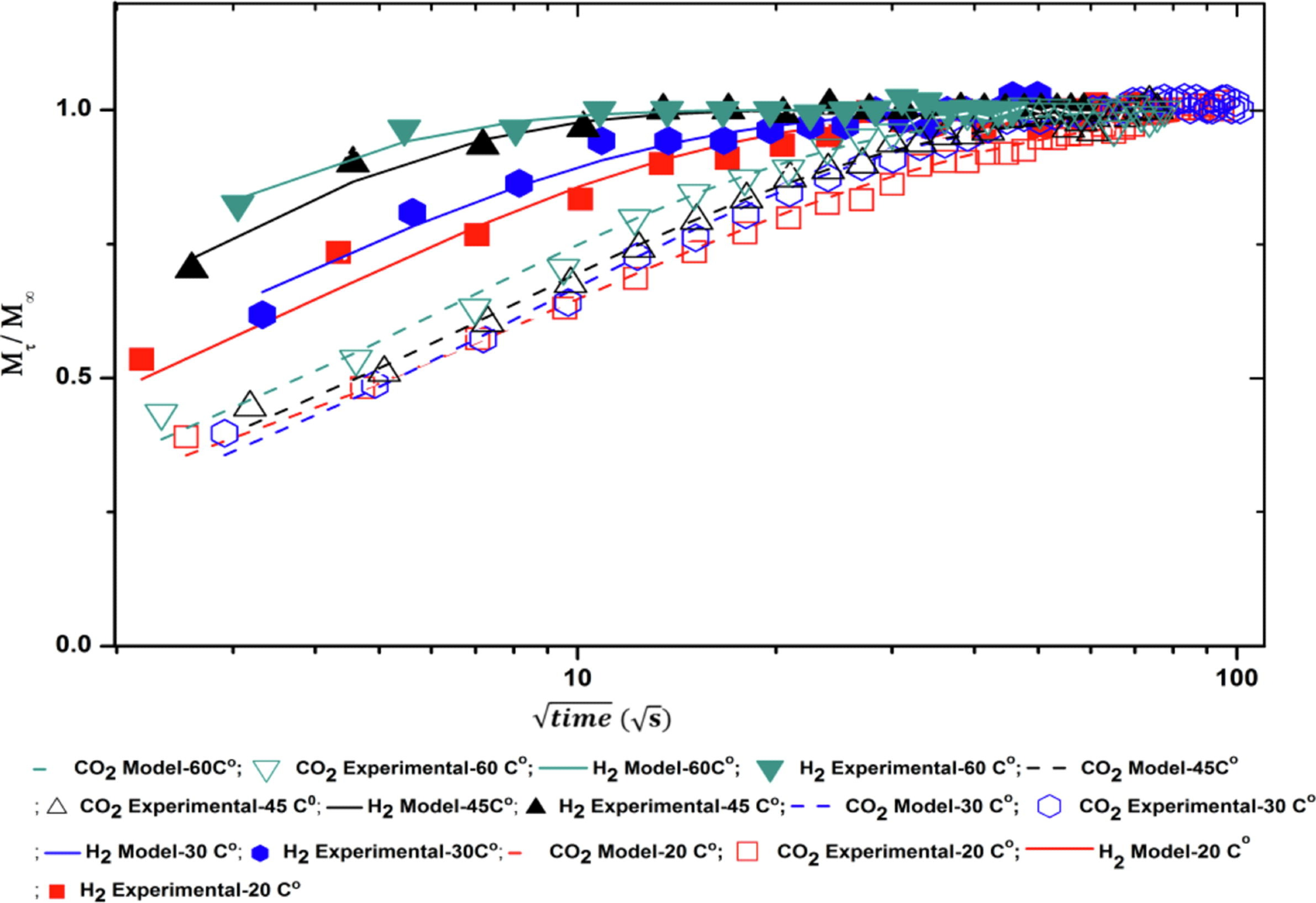
Figure. 2. Comparing H2 and CO2 adsorption rate parameters: Adsorption rate profile for H2 and CO2 as a function of temperature (equilibrium pressures were in the range of 12.78–13.03 bar in all H2 and CO2 kinetics tests). Image Credit: Keshavarz, et al., 2022
Moreover, in all diffusion tests (that is, for both H2 and CO2), β was nearly constant (β = 0.36 ± 7.5%) (see Table 2 and Figure 3).
Also, 1/t0, and, as a result, D—the diffusion coefficient, increased as temperature increased for both gases (see Figure 4 and Table 2).
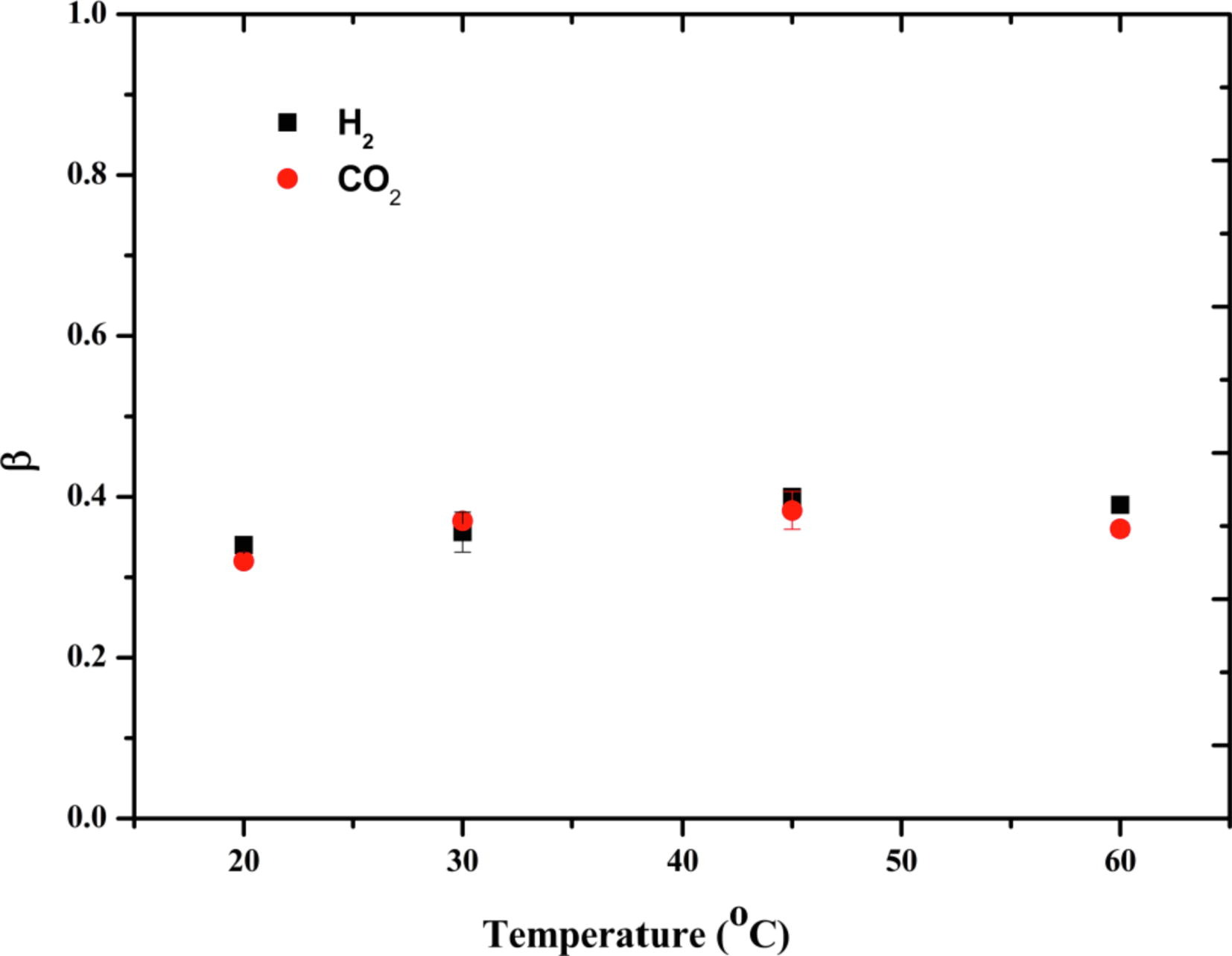
Figure. 3. β values for H2 and CO2 tests at different temperatures. Image Credit: Keshavarz, et al., 2022
Moreover, it is well-known that gas adsorption (along with that of CO2 and H2) decreases with an increase in temperature (see Figure 4).
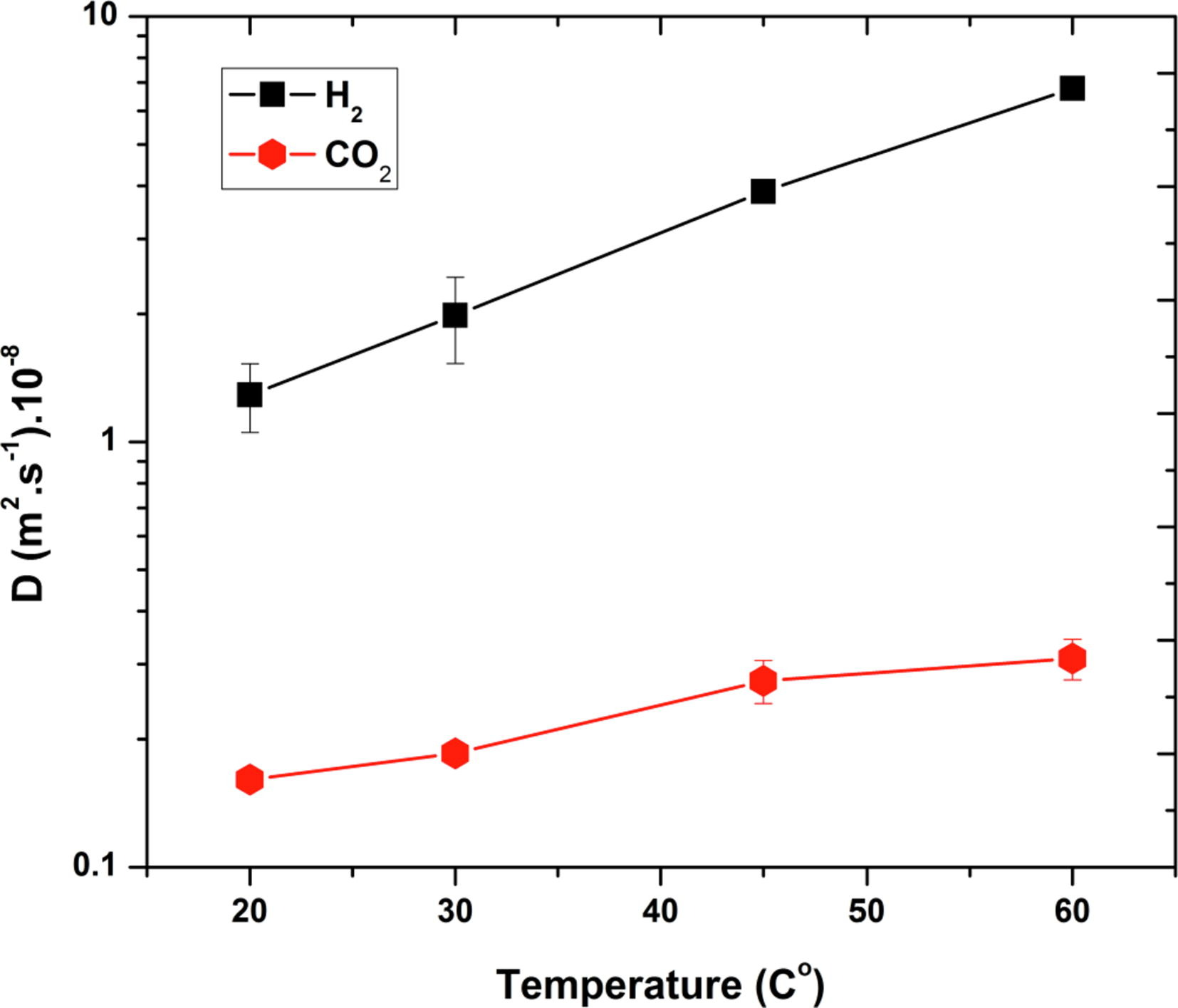
Figure. 4. H2 and CO2 diffusion coefficients at different temperatures. Image Credit: Keshavarz, et al., 2022
Furthermore, increasing the temperature increased the H2–CO2 diffusion coefficient ratio, whereas the H2–CO2 adsorption ratio stayed the same (see Figure 5).
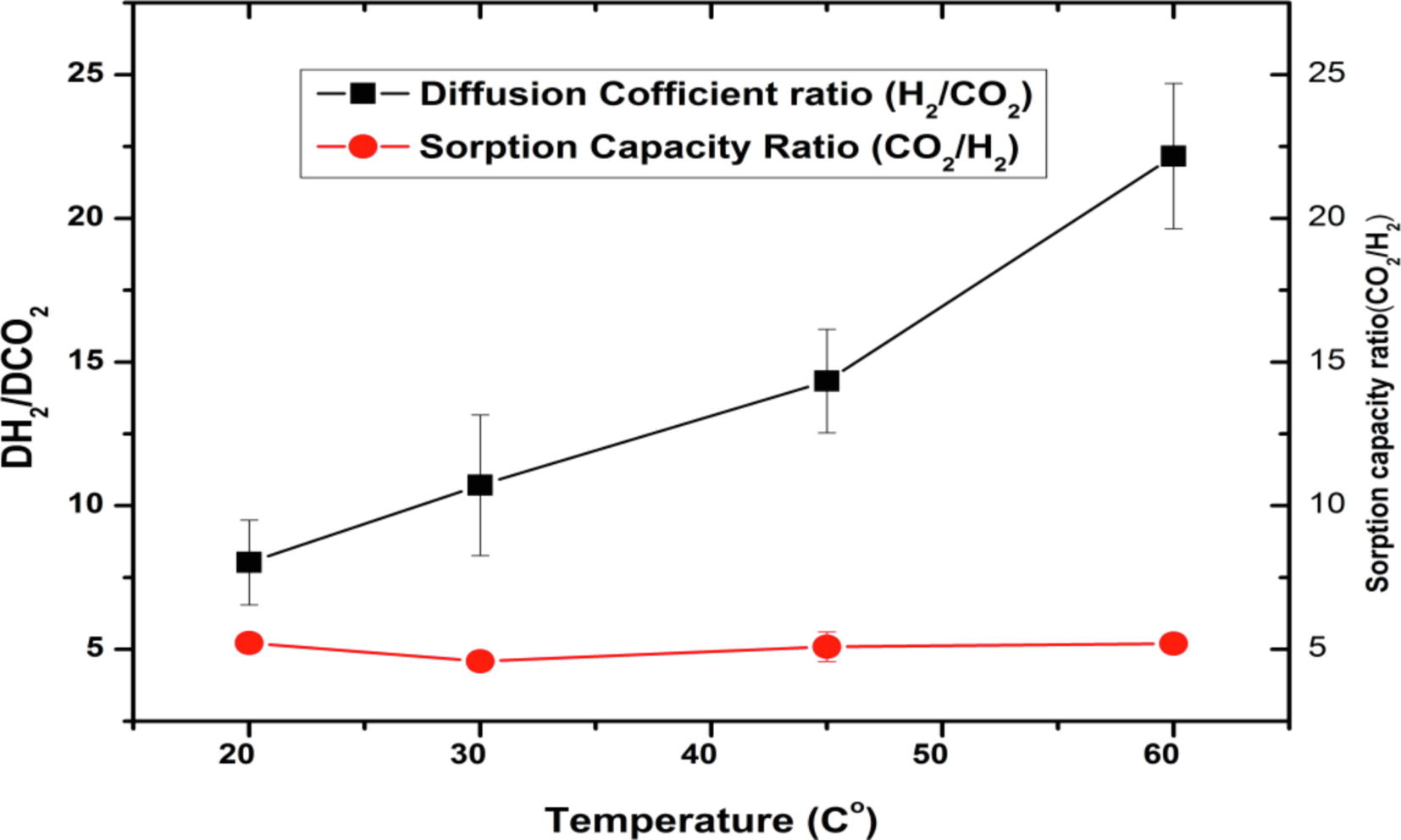
Figure. 5. Diffusion coefficient ratio (H2/CO2) and sorption capacity ratio (CO2/H2) at different temperatures. Image Credit: Keshavarz, et al., 2022
It is to be noted that for all tested temperatures, CO2 adsorption ability was considerably higher than that of H2 (by five times approximately), whereas adsorption capability decreased slightly for both gases with an increase in temperature (see Figure 6).
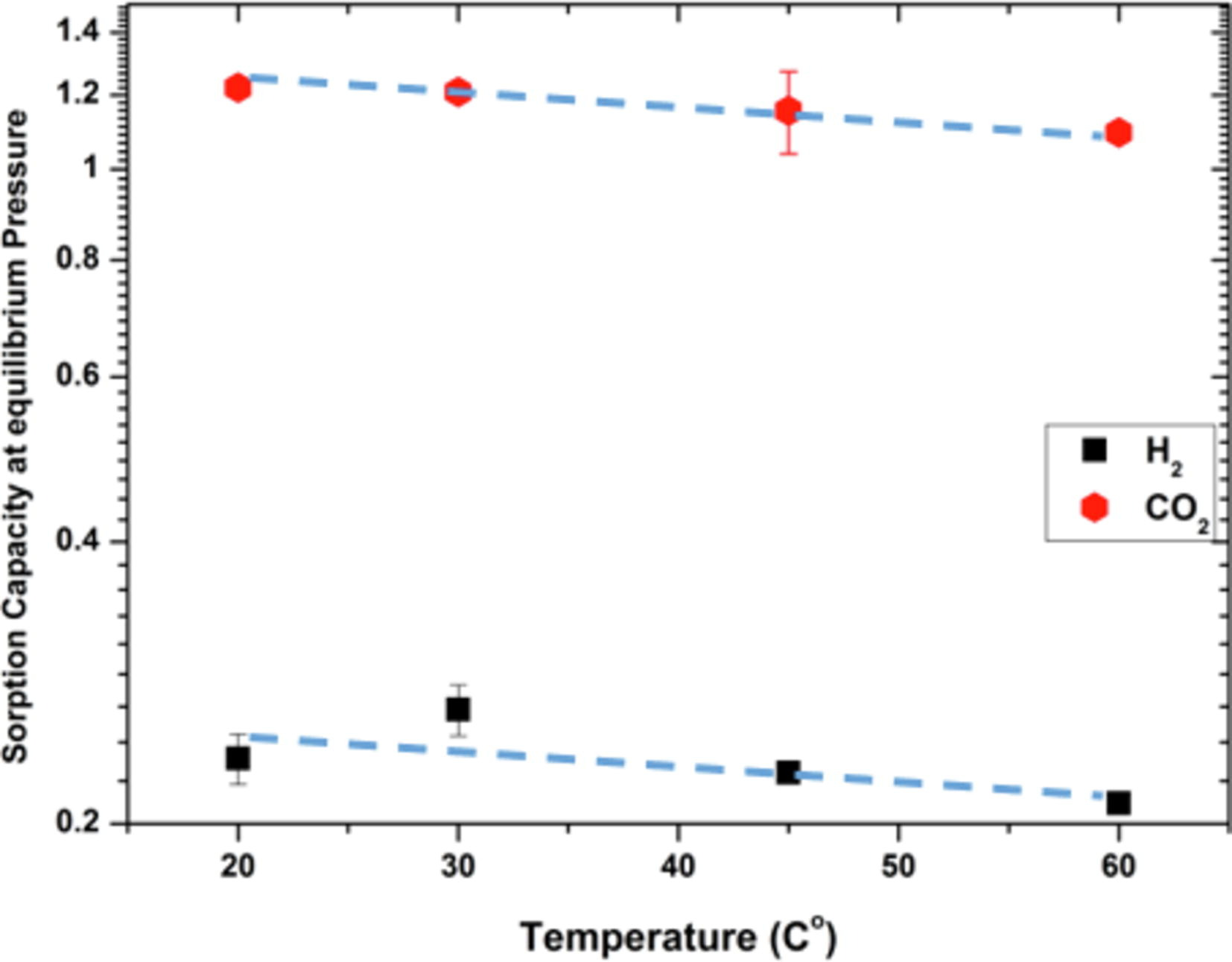
Figure. 6. H2 and CO2 adsorption capacities at equilibrium pressure (12.78–13.03 bar) as a function of temperature. Image Credit: Keshavarz, et al., 2022
Conclusion
Even though gas diffusion in coal is a well-established phenomenon, there is a severe lack of data for hydrogen diffusion in coal, majorly owing to the novelty of the geologic hydrogen storage concept.
Hence, in this study, depending on already reported gas diffusion measurements in coal, the researchers quantified kinetic adsorption profiles of H2 for four different temperatures (20 °C, 30 °C, 45 °C, and 60 °C) and equilibrium pressures of ∼13 bar on an Australian anthracite coal sample at isothermal conditions. CO2 diffusion coefficients were quantified for the same sample at similar equilibrium pressure and temperatures for comparison.
The results reveal that a higher temperature results in a higher rate of H2 and CO2 adsorption, but leads to a lower gas adsorption capacity. They also show that H2 diffusion coefficients are larger than the equivalent CO2 diffusion coefficients with one order of magnitude. The H2−CO2 diffusion coefficient ratio improved from 10 at 20 °C to 22 at 60 °C.
Furthermore, CO2 adsorption capacities are approximately five times larger than the equivalent H2 adsorption capacities, at all studied temperatures and equilibrium pressure (∼13 bar). In addition, H2 kinetic research is underway to find the coal characteristics’ effect on H2 diffusion in coal.
Journal Reference:
Keshavarz, A., Abid, H., Ali, M., Iglauer, S. (2022) Hydrogen diffusion in coal: Implications for hydrogen geo‐storage. Journal of Colloid and Interface Science. Volume 608, Part 2, P. 1457–1462. Available Online: https://www.sciencedirect.com/science/article/pii/S0021979721017276.
References and Further Reading
- Hanley, E. S., et al. (2018) The role of hydrogen in low carbon energy futures–A review of existing perspectives. Renewable and Sustainable Energy Reviews, 82, pp. 3027–3045. doi.org/10.1016/j.rser.2017.10.034
- Tarkowski, R & Czapowski, G (2018) Salt domes in Poland–potential sites for hydrogen storage in caverns. International Journal of Hydrogen Energy, 43 (46), pp. 21414–21427. doi.org/10.1016/j.ijhydene.2018.09.212
- Zhang, F., et al. (2016) The survey of key technologies in hydrogen energy storage. International Journal of Hydrogen Energy, 41 (33), pp. 14535–14552. doi.org/10.1016/j.ijhydene.2016.05.293.
- Berta, M., et al. (2018) Geochemical effects of millimolar hydrogen concentrations in groundwater: an experimental study in the context of subsurface hydrogen storage. Environmental Science & Technology, 52 (8), pp. 4937–4949. doi.org/10.1021/acs.est.7b05467
- Flesch, S., et al. (2018) Hydrogen underground storage—Petrographic and petrophysical variations in reservoir sandstones from laboratory experiments under simulated reservoir conditions. International Journal of Hydrogen Energy, 43 (45), pp. 20822–20835. doi.org/10.1016/j.ijhydene.2018.09.112
- Heinemann, N., et al. (2018) Hydrogen storage in porous geological formations–onshore play opportunities in the midland valley (Scotland, UK). International Journal of Hydrogen Energy, 43 (45), pp. 20861–20874. doi.org/10.1016/j.ijhydene.2018.09.149
- Lord, A. S., et al. (2014) Geologic storage of hydrogen: Scaling up to meet city transportation demands. International Journal of Hydrogen Energy, 39 (28), pp. 15570–15582. doi.org/10.1016/j.ijhydene.2014.07.121
- Shi, Z., et al. (2020) Impacts of the subsurface storage of natural gas and hydrogen mixtures. International Journal of Hydrogen Energy, 45(15), pp. 8757–8773. doi.org/10.1016/j.ijhydene.2020.01.044.
- Yekta, A. E., et al. (2018) Determination of hydrogen–water relative permeability and capillary pressure in sandstone: application to underground hydrogen injection in sedimentary formations. Transport in Porous Media, 122 (2), pp. 333–356. doi.org/10.1007/s11242-018-1004-7
- Iglauer, S., et al. (2021) Hydrogen Adsorption on Sub-Bituminous Coal: Implications for Hydrogen Geo-Storage. Geophysical Research Letters, 48 (10), p. e2021GL092976. doi.org/10.1029/2021GL092976
- Iglauer, S., et al. (2021) Hydrogen Wettability of Sandstone Reservoirs: Implications for Hydrogen Geo-Storage. Geophysical Research Letters, 48 (3), p. e2020GL090814. doi.org/10.1029/2020GL090814
- Hashemi, L., et al. (2021) Pore-scale modelling and sensitivity analyses of hydrogen-brine multiphase flow in geological porous media. Scientific Reports, 11 (1), pp. 1–13. doi.org/10.1038/s41598-021-87490-7
- Hashemi, L., et al. (2021) Contact Angle Measurement for Hydrogen/Brine/Sandstone System Using Captive-Bubble Method Relevant for Underground Hydrogen Storage. Advances in Water Resources, 154, p. 103964. doi.org/10.1016/j.advwatres.2021.103964.
- Pan, B., et al. (2021) Rock-fluid interfacial tension at subsurface conditions: Implications for H2, CO2 and natural gas geo-storage. International Journal of Hydrogen Energy, 46 (50), pp. 25578–25585.
- Pan, B., et al. (2021) Underground hydrogen storage: Influencing parameters and future outlook. Advances in Colloid and Interface Science, 294, p. 102473, doi.org/10.1016/j.cis.2021.102473.
- Ali, M., et al. (2021) Influence of pressure, temperature and organic surface concentration on hydrogen wettability of caprock; implications for hydrogen geo-storage. Energy Reports, 7, pp. 5988–5996. doi.org/10.1016/j.egyr.2021.09.016
- Keshavarz, A., et al. (2017) Effect of maceral composition and coal rank on gas diffusion in Australian coals. International Journal of Coal Geology, 173, pp. 65–75. doi.org/10.1016/j.coal.2017.02.005
- Buzek, F & Lnenickova, Z (2010) Temperature programmed desorption of coal gases–Chemical and carbon isotope composition. Fuel, 89 (7), pp. 1514–1524. doi.org/10.1016/j.fuel.2009.09.020
- Staib, G., et al. (2015) Dispersive diffusion of gases in coals. Part I: Model development. Fuel, 143, pp. 612–619. doi.org/10.1016/j.fuel.2014.11.086
- Staib, G., et al. (2015) Dispersive diffusion of gases in coals. Part II: An assessment of previously proposed physical mechanisms of diffusion in coal. Fuel, 143, pp. 620–629. doi.org/10.1016/j.fuel.2014.11.087
- Kiani, A., et al. (2018) Gas sorption capacity, gas sorption rates and nanoporosity in coals. International Journal of Coal Geology, 200, pp. 77–86. doi.org/10.1016/j.coal.2018.10.012.
- Kiani, A., et al. (2019) The use of infrared spectroscopy to determine methane emission rates from coals at atmospheric pressures. Energy Fuels, 33 (1), pp. 238–247. doi.org/10.1021/acs.energyfuels.8b03547
- Remner, D. J., et al. (1986) A parametric study of the effects of coal seam properties on gas drainage efficiency. SPE Reservoir Engineering, 1 (06), pp. 633–646. doi.org/10.2118/13366-PA
- Charrière, D., et al. (2010) Effect of pressure and temperature on diffusion of CO2 and CH4 into coal from the Lorraine basin (France). International Journal of Coal Geology,81 (4), pp. 373–380. doi.org/10.1016/j.coal.2009.03.007
- Wang, Z., et al. (2015) Physical simulation of temperature influence on methane sorption and kinetics in coal: Benefits of temperature under 273.15 K. Fuel, 158, pp. 207–216. https://doi.org/10.1016/j.fuel.2015.05.011
- Yue, G., et al. (2015) Physical simulation of temperature influence on methane sorption and kinetics in coal (II): temperature evolvement during methane adsorption in coal measurement and modelling. Energy Fuels, 29 (10), pp. 6355–6362. doi.org/10.1021/acs.energyfuels.5b01637
- Brush, Stephen G. (2013). Kinetic Theory: The Chapman–Enskog Solution of the Transport Equation for Moderately Dense Gases. Vol. 3. Elsevier,
- Brush, S. G (2003) Kinetic Theory of Gases, The: An Anthology of Classic Papers With Historical Commentary, 1. World Scientific.
- Kentish, S., et al. (2008) Carbon dioxide separation through polymeric membrane systems for flue gas applications. Recent Patents on Chemical Engineering, 1 (1) (2008), pp. 52–66. doi.org/10.2174/2211334710801010052
- Kadoura, A., et al. (2016) Molecular dynamics simulations of carbon dioxide, methane, and their mixture in montmorillonite clay hydrates. The Journal of Physical Chemistry C, 120 (23), pp. 12517–12529. doi.org/10.1021/acs.jpcc.6b02748
- Teng, Y., et al. (1997) Study of the fundamentals of adsorption systems. Applied Thermal Energy, 17 (4), pp. 327–338. doi.org/10.1016/S1359-4311(96)00039-7
- Wang, K., et al. (2017) Investigation of gas pressure and temperature effects on the permeability and steady-state time of Chinese anthracite coal: An experimental study. Journal of Natural Gas Science and Engineering, 40, pp. 179–188. doi.org/10.1016/j.jngse.2017.02.014
- Zhang, Y., et al. (2015) Pure methane, carbon dioxide, and nitrogen adsorption on anthracite from China over a wide range of pressures and temperatures: Experiments and modelling. RSC Advances, 5 (65), pp. 52612–52623. doi.org/10.1039/C5RA05745K
- Buckingham, A. D., et al. (1968) Quadrupole moments of some simple molecules. Journal of American Chemical Society, 90 (12), pp. 3104–3107. doi.org/10.1021/ja01014a023
- Buckingham, A. D (1959) Molecular quadrupole moments. Quarterly Reviews, Chemical Society, 13 (3), p. 183. doi.org/10.1039/qr9591300183.
- Ralser, S., et al. (2016) Experimental evidence for the influence of charge on the adsorption capacity of carbon dioxide on charged fullerenes. PCCP, 18 (4), pp. 3048–3055. doi.org/10.1039/C5CP06587A
- Abid, H. R., et al. (2021) Hussein Rasool Abid, Stefan Iglauer, Ahmed Al-Yaseri, Alireza Keshavarz. Drastic enhancement of CO2 adsorption capacity by negatively charged sub-bituminous coal. Energy, 233, p. 120924. doi.org/10.1016/j.energy.2021.120924.
- Plerdsranoy, P., et al. (2021) Hydrogen adsorption of O/N-rich hierarchical carbon scaffold decorated with Ni nanoparticles: Experimental and computational studies. International Journal of Hydrogen Energy, 46 (7), pp. 5427–5440. doi.org/10.1016/j.ijhydene.2020.11.042.
- Gregory N., et al. (2015) Comparing the porosity and surface areas of coal as measured by gas adsorption, mercury intrusion and SAXS techniques. Fuel, pp. 293–304. doi.org/10.1016/j.fuel.2014.10.046
- Arif, M., et al. (2017) Influence of surface chemistry on interfacial properties of low to high rank coal seams. Fuel, 194, pp. 211–221. doi.org/10.1016/j.fuel.2017.01.027.
- Fujii, A., et al. (2002) Direct observation of weak hydrogen bonds in microsolvated phenol: Infrared spectroscopy of OH stretching vibrations of phenol− CO and − CO2 in S0 and D0. The Journal of Physical Chemistry A, 106 (43), pp. 10124–10129.
- Raveendran, P., et al. (2005) Polar attributes of supercritical carbon dioxide. Accounts of Chemical Research., 38 (6), pp. 478–485.
- Awoyemi A (2011) Understanding the adsorption of polycyclic aromatic hydrocarbons from aqueous phase onto activated carbon.
- Nguyen-Thuy, T., et al. (2020) Hydrogen adsorption mechanism of MOF-74 metal–organic frameworks: an insight from first principles calculations. RSC Advances, 10 (72), pp. 43940–43949.
- Shen, F., et al. (2019) Roles of Oxygen Functional Groups in Hydrogen Sulfide Adsorption on Activated Carbon Surface: A Density Functional Study. Industrial & Engineering Chemistry Research, 58 (14), pp. 5526–5532.
- Busch, A & Gensterblum, Y (2011) CBM and CO2-ECBM related sorption processes in coal: a review. International Journal of Coal Geology, 87 (2), pp. 49–71.
- Muhammad Ali, et al (2021) Influence of Organic Molecules on Wetting Characteristics of Mica/H2/Brine Systems: Implications for Hydrogen Structural Trapping Capacities. Journal of Colloid and Interface Science. doi.org/10.1016/j.jcis.2021.10.080.