A huge portion of the world’s population does not have access to electricity. Standard photovoltaic (PV) cells could provide a renewable off-grid source of electricity—however—they can only generate electricity during the day and not at night.

Image Credit: yanikap/Shutterstock.com
While there have been diverse conceptual suggestions and practical implementations of energy harvesting from a PV cell’s radiative cooling at night, the power density obtained is quite low.
Researchers have built a thermoelectric generator that generates electricity from the difference in temperature between the PV cell and the surrounding environment. This research has been published in Applied Physics Letters.
With a clear night sky and a 100 mV open-circuit voltage, researchers obtain 50 mW/m2 nighttime power generation, which is orders of magnitude greater than earlier performances.
Producing power during the night in many rural regions relying on mini-grid or off-grid systems sometimes demands major additional battery storage installation, which adds significant system complications.
Heat transfer from a hot source to a cool sink is required to generate work from heat, according to thermodynamics. During the day, a PV cell acts as a heat engine, converting solar energy shining on the PV cell into electrical power utilizing the Sun as the heat source and the Earth’s ambient surrounds as the cold sink. Radiative heat flux from Earth to outer space occurs in addition to solar irradiation.
Since the Earth’s atmosphere is clear in the mid-infrared wavelength range, such outward heat transfer is conceivable. As it must, on average, balance the heat flow from the incoming solar flux for Earth to sustain its temperature, the magnitude of such departing heat flow is very significant.
As a result, every item on Earth that has access to the sky can radiate heat into space, providing a method for radiative cooling. The recent focus on accomplishing cooling in diverse materials and incorporation in energy-related activities, such as building cooling, has sparked a lot of interest in this type of radiative cooling effect.
The PV-TEG device’s operating principle is depicted in Figure 1 from the standpoint of the PV cell’s energy balance at night.

Figure 1. Nighttime power generation from radiative cooling of a PV cell. (a) Schematic showing the energy balance of the PV cell and (b) thermal circuit model of the PV-TEG device. Image Credit: Assawaworrarit, et al., 2022
The PV-TEG device’s design drawing and completed prototype are shown in Figures 2(a) and 2(b). The device is built around a SunPower C-60 monocrystalline silicon solar cell with an A = 153 cm2 surface area.
Researchers designed an air-insulated chamber around the PV cell with an entrance at the bottom for heat sink connection to limit parasitic heat flow with the ambient. Low-density polyethylene (LDPE) film is used to cover the top of the chamber, which is translucent for both visual and mid-infrared wavelength ranges.
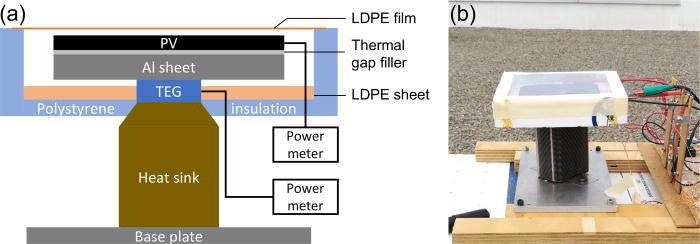
Figure 2. Design and prototype of a PV-TEG device. (a) Design drawing and (b) constructed prototype. Image Credit: Assawaworrarit, et al., 2022

Figure 3. Multi-day temperature and power measurements. (a) Temperature measurements. (b) PV power generation. (c) TEG power generation. Note the different scales used for daytime and nighttime. (d) Snapshot of the TEG’s current-voltage–power relationship at 8:50 pm on October 11. Image Credit: Assawaworrarit, et al., 2022
The power and temperature readings are shown in Figure 3. Temperature measurements (Figure 3(a)) reveal that the PV cell warms up during the day because of absorbing solar radiation. The PV cell reaches a peak temperature difference of roughly 15 °C above ambient around midday. Figure 3(b) shows how the measured daytime temperature difference correlates with PV cell power.
The TEG power values are shown in Figure 3(c).
For the nights of October 10 and 11, when the sky is clear, Figure 4 indicates that the recorded nighttime power generation coincides well with the prediction models.

Figure 4. Comparison between modeled nighttime power generation and actual measurements (line) during the nights of October 10 (a) and October 11 (b). Shaded areas represent model predictions using the correlation model, which provides a range of εatm. Image Credit: Assawaworrarit, et al., 2022
Conclusion
In conclusion, researchers have developed a PV-TEG system that can prolong PV cell power generation into the night by employing radiative cooling while also supplying extra power during the day. Based on region and sky quality, nocturnal power generation ranges from 10 to 100 mW/m2.
In off-grid and mini-grid situations, where PV cell installations are becoming more prevalent, the solution can supply midnight backup lighting and electricity. Since thermoelectric generators have a long service life, the proposed system may have lower long-term maintenance costs than battery storage.
The documented power density is already attracting attention for applications such as nighttime lighting. The system can also power sensors in far-flung places, lowering or eliminating the need for battery storage.
Journal Reference:
Assawaworrarit, S., Omair, Z., Fan, S. (2022) Nighttime electric power generation at a density of 50 mW/m2 via radiative cooling of a photovoltaic cell. Applied Physics Letters, 120(14), p. 143901. Available Online: https://aip.scitation.org/doi/10.1063/5.0085205.
References and Further Reading
- Comello, S. D., et al. (2006) Enabling Mini-Grid Development in Rural India. World Development, 93, pp. 94–107. doi.org/10.1016/j.worlddev.2016.12.029.
- Sandwell, P., et al. (2016) Off-grid solar photovoltaic systems for rural electrification and emissions mitigation in India. Solar Energy Materials and Solar Cells, 156, pp. 147–156. doi.org/10.1016/j.solmat.2016.04.030.
- Deichmann, U., et al. (2011) The economics of renewable energy expansion in rural Sub-Saharan Africa. Energy Policy, 39, pp. 215–227. doi.org/10.1016/j.enpol.2010.09.034.
- Granqvist, C G & Hjortsberg, A (1981) Radiative cooling to low temperatures: General considerations and application to selectively emitting SiO films. Journal of Applied Physics, 52(6), p. 4205. doi.org/10.1063/1.329270.
- Zhao, D., et al. (2019) Radiative sky cooling: Fundamental principles, materials, and applications. Applied Physics Reviews, 6(2), p. 021306. doi.org/10.1063/1.5087281.
- Zhao, B., et al. (2019) Radiative cooling: A review of fundamentals, materials, applications, and prospects. Applied Energy, 236, pp. 489–513. doi.org/10.1016/j.apenergy.2018.12.018.
- Bartoli, B., et al. (1977) Nocturnal and diurnal performances of selective radiators. Applied Energy, 3(4), pp. 267–286. doi.org/10.1016/0306-2619(77)90015-0.
- Gentle, A R & Smith, G B (2010) Radiative Heat Pumping from the Earth Using Surface Phonon Resonant Nanoparticles. Nano Letters, 10(2), pp. 373–379. doi.org/10.1021/nl903271d.
- Rephaeli, E., et al. (2013) Ultrabroadband Photonic Structures To Achieve High-Performance Daytime Radiative Cooling. Nano Letters, 13(4), pp. 1457–1461. doi.org/10.1021/nl4004283.
- Raman, A. P., et al. (2014) Passive radiative cooling below ambient air temperature under direct sunlight. Nature, 515, pp. 540–544. doi.org/10.1038/nature13883.
- Hossain, M & Gu, M (2016) Radiative Cooling: Principles, Progress, and Potentials. Advance Science, 3(7), p. 1500360. doi.org/10.1002/advs.201500360.
- Zeyghami, M., et al. (2018) A review of clear sky radiative cooling developments and applications in renewable power systems and passive building cooling. Solar Energy Materials and Solar Cells, 178, pp. 115–128. doi.org/10.1016/j.solmat.2018.01.015.
- Bagiorgas, H S & Mihalakakou, G (2008) Experimental and theoretical investigation of a nocturnal radiator for space cooling. Renewable Energy, 33(6), pp. 1220–1227. doi.org/10.1016/j.renene.2007.04.015.
- Zhang, S & Niu, J (2012) Cooling performance of nocturnal radiative cooling combined with microencapsulated phase change material (MPCM) slurry storage. Energy Building, 54, pp. 122–130. doi.org/10.1016/j.enbuild.2012.07.041.
- Byrnes, S. J., et al. (2014) Harvesting renewable energy from Earth’s mid-infrared emissions. Processing of the National Academy of Sciences of the USA, 111(11), pp. 3927–3932. doi.org/10.1073/pnas.1402036111.
- Buddhiraju, S., et al. (2018) Thermodynamic limits of energy harvesting from outgoing thermal radiation. Processing of the National Academy of Sciences of the USA, 115(16), pp. E3609–E3615. doi.org/10.1073/pnas.1717595115.
- Li, W., et al. (2020) Thermodynamic limits for simultaneous energy harvesting from the hot sun and cold outer space. Light: Science & Applications, 9, p. 68. doi.org/10.1038/s41377-020-0296-x.
- Fan, L., et al. (2020). Maximal nighttime electrical power generation via optimal radiative cooling. Optics Express, 28(17), pp. 25460–25470. doi.org/10.1364/OE.397714.
- Lorenzi, B., et al. (2020) Self-powered broadband photo-detection and persistent energy generation with junction-free strained Bi2Te3 thin films. Optics Express, 28(19), pp. 27644–27656. doi.org/10.1364/OE.399040.
- Santhanam, P & Fan, S (2016) Thermal-to-electrical energy conversion by diodes under negative illumination. Physical Review B, 93, p. 161410. doi.org/10.1103/PhysRevB.93.161410.
- Liao, T., et al. (2017) Negative illumination thermoradiative solar cell. Optics Letters, 42(16), pp. 3236–3238. doi.org/10.1364/OL.42.003236.
- Ono, M., et al. (2019) Experimental demonstration of energy harvesting from the sky using the negative illumination effect of a semiconductor photodiode. Applied Physics Letters, 114(16), p. 161102. doi.org/10.1063/1.5089783.
- Raman, A. P., et al. (2019) Generating Light from Darkness. Joule, 3(11), pp. 2679–2686. doi.org/10.1016/j.joule.2019.08.009.
- Li, G., et al. (2018) A review of solar photovoltaic-thermoelectric hybrid system for electricity generation. Energy, 158, pp. 41–58. doi.org/10.1016/j.energy.2018.06.021.
- Van Sark, W G J H M (2011) Feasibility of photovoltaic – Thermoelectric hybrid modules. Applied Energy, 88(8), pp. 2785–2790. doi.org/10.1016/j.apenergy.2011.02.008.
- Rejeb, O., et al. (2020) Optimization and performance analysis of a solar concentrated photovoltaic-thermoelectric (CPV-TE) hybrid system. Renewable Energy, 152, pp. 1342–1353. doi.org/10.1016/j.renene.2020.02.007.
- Zhao, B., et al. (2021) Is it possible for a photovoltaic-thermoelectric device to generate electricity at night? Solar Energy Materials and Solar Cells, 228, p. 111136. doi.org/10.1016/j.solmat.2021.111136.
- Li, W., et al. (2018) Photonic thermal management of coloured objects. Nature Communication, 9, p. 4240. doi.org/10.1038/s41467-018-06535-0.
- II-VI Marlow Inc, (2014) Technical data sheet for TG12-4.
- Berdahl, P & Fromberg, R (1982) The thermal radiance of clear skies. Solar Energy, 29(4), pp. 299–314. doi.org/10.1016/0038-092X(82)90245-6.
- Li, W., et al. (2017) A Comprehensive Photonic Approach for Solar Cell Cooling. ACS Photonics, 4(4), pp. 774–782. doi.org/10.1021/acsphotonics.7b00089